the Creative Commons Attribution 4.0 License.
the Creative Commons Attribution 4.0 License.
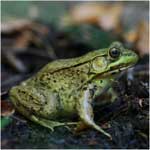
Tracking ranavirus infections: an integrative review of epidemiological research on pathogen dynamics in anurans
Davi dos Santos Rodrigues
Rita de Cássia Carvalho Maia
Geraldo Jorge Barbosa de Moura
Ricardo Luiz Moro de Sousa
José Wilton Pinheiro Junior
Emerging infectious diseases have contributed to the decline in amphibian species around the world. Among them, ranavirus infections have major importance, as they significantly impact biodiversity and frog rearing. This study aims to conduct an integrative review of the recent literature, including epidemiological data on the distribution, prevalence, and circulating species and strains, to identify gaps that may still exist and suggest potential directions for future research. We synthesized data collected from 68 articles found in two scientific databases (Scopus and PubMed), and we provide a situational update on ranavirus infections in anurans. We found relevant reports confirming the high prevalence of this pathogen and the risk it poses, including to endangered species. However, there is still information to be clarified regarding its distribution in different territories and the identification of the species involved in infections.
- Article
(921 KB) - Full-text XML
- BibTeX
- EndNote
Amphibians are a diverse and cosmopolitan class of vertebrates, comprising over 8000 species (Frost, 2024). Despite this diversity, amphibians are at risk of extinction. According to the Red List of Threatened Species of the International Union for the Conservation of Nature (IUCN), 41 % of amphibian species are at risk of disappearing (IUCN, 2023). Several factors are behind this decline, including climate change, deforestation, habitat fragmentation (Santi and Corrêa, 2018), and the introduction of alien species (Kraus, 2015). Moreover, the occurrence of infectious diseases significantly impacts the numbers of many of these species (Daszak et al., 2003). Ranaviruses, viruses belonging to the Iridoviridae family (Chinchar et al., 2017) that infect fish, amphibians, and reptiles (Brenes et al., 2014), are among the most important emerging pathogens.
Ranaviruses are large viruses (150 to 200 nm in diameter), with a genome consisting of a double strand of DNA (dsDNA); are covered by an inner membrane, which in turn is covered by a capsid of icosahedral symmetry; and can be enveloped by an outer envelope (in the case of species that bud from the host cell's plasma membrane) or not. These morphological characteristics are common to all members of this taxon (Chinchar et al., 2017). Seven species are currently recognized: Ambystoma tigrinum virus (ATV), Common midwife toad virus (CMTV), Epizootic haematopoietic necrosis virus (EHNV), Santee-Cooper virus (Largemouth bass virus, LMBV), Singapore grouper iridovirus (SGIV), European North Atlantic ranavirus (LfRV), and Frog virus 3 (FV3) (Jancovich et al., 2012). The main species that affect amphibians are CMTV, ATV and FV3, and studies have shown that anurans and urodeles are more susceptible to ATV and FV3, respectively (Schock et al., 2008).
Ranaviruses are considerably resistant in natural environments: for example, a laboratory study showed that FV3 persisted outside a host at low temperatures (4 °C) for up to 102 d in filtered river water and 57 d in unfiltered water (Nazir et al., 2012). In addition, these viruses can also remain in sediments, where they are able to conserve their infecting capacity, under the same temperature range (Munro et al., 2016). Virions are inactivated by acidic (< 3.0) and alkaline (> 11.0) pH, exposure to UV irradiation, and high temperature (> 55 °C for 30 min). In addition, ranaviruses are sensitive to the action of disinfectants such as chlorhexidine, sodium hypochlorite, and potassium compounds (Bryan et al., 2009).
The first description of ranavirus infection occurred in the 1960s; since then, several die-off events have been recorded. These viruses have been identified as causing epizootic outbreaks worldwide (Gray and Miller, 2013; Maclachlan; Dubovi, 2016), with cases reported in several countries (Duffus et al., 2015). The disease has a high mortality rate and can reach 100 % death rates in infected populations (World Organisation for Animal Health, 2021), thus bringing significant losses to aquaculture and biodiversity: there are scientific reports that reveal the decline in amphibian species in the wild due to infections by ranavirus in Europe (Price et al., 2014), as well as mass mortalities of animals in the wild (Miaud et al., 2016) and captivity (Une et al., 2014). For these reasons, ranavirus infection was listed as a notifiable disease by the World Organisation for Animal Health (WOAH) in 2009, although it is not a zoonotic disease (Black et al., 2017).
The ecology of ranaviruses involves a complex chain of interaction between host species, transmission routes, environmental persistence, stressors, and the immunity of the host individual (Gray et al., 2012). The virus is transmitted horizontally. A high population density can facilitate transmission between individuals, through direct contact between healthy and sick animals. In addition, natural behaviors among amphibians, such as predation (cannibalism and necrophagy), are risk factors for direct transmission of the virus (Harp and Petranka, 2006; Latney and Klaphake, 2013). The virus can also be dispersed through water, soil, and sediment and infect other individuals indirectly (Nazir et al., 2012).
Affected animals can show symptoms such as altered buoyancy, erratic swimming, lethargy, edema, erythema, and skin ulcers that can develop into skin necrosis with loss of extremities (Forzán et al., 2015; Miller et al., 2007; Stöhr et al., 2013). Despite the variety of clinical signs, some animals may harbor a subclinical but transmissible infection of the virus (Brunner et al., 2019); these carriers contribute to the dispersal of the pathogen, especially when considering the wild-animal trade, which some authors point to as a “source of pathogen pollution” (Picco and Collins, 2008).
In the last few years, there has been a significant increase in research involving ranaviruses, evidenced by the growth in the number of articles published on the subject (Wirth et al., 2021). Anurans are the main models used to evaluate important aspects of the infection, such as immunity, pathogenesis (Forzán et al., 2017; Morales et al., 2010), diagnostic techniques (Ford et al., 2022), transmission potential (Cunningham et al., 2007), and environmental drivers (Hall et al., 2020). Furthermore, since ranaviruses can accelerate the process of extinction of threatened species, the risk of disappearance of anurans is concerning from a conservation perspective (Earl et al., 2016). Given the amount of available information on ranavirus infection in anurans, this study aims at conducting an integrative literature review to collect and synthesize data published between 2013 and 2022. We intend to provide a situational update on the epidemiology of ranavirus infections in anurans worldwide and identify possible gaps in knowledge that could be the subject of future research.
This research consisted of an integrative review to analyze data on the prevalence, geographical distribution, and diversity of ranavirus species infecting anurans worldwide. The search criteria included studies published within a 10-year period (2013–2022). The search for articles was conducted in two bibliographic databases: Scopus and PubMed. The search terms were applied using Boolean operators: ranavirus AND infection AND (anurans OR amphibian) AND (epidemiology OR prevalence OR occurrence). We predefined the inclusion and exclusion criteria for the articles (Table 1) and applied them for screening and subsequent selection.
The search in the two databases yielded 921 articles. After retrieval, the titles and abstracts were read. A total of 746 articles did not meet the established criteria and were excluded. The articles that passed the screening were read in full; the inclusion and exclusion criteria were also considered. Those that did not provide the full text for reading were rejected (3 articles). In the end, 68 articles were selected (Fig. 1).
Importantly, when the study did not provide the prevalence of Ranavirus, we calculated it if access to the data needed to do so for the species of interest were available. In articles where the prevalence was defined for other orders of anurans (e.g., Caudata), a prevalence ratio was conducted considering only anurans. Studies wherein this information was impossible to retrieve were discarded. To calculate prevalence, the equation comprising the ratio between the total number of animals detected as positive and the total number of animals tested was applied. This calculation follows the prevalence principle described by Lima et al. (2018), in which it is measured by dividing the number of known cases of a given disease by the number of individuals in the population at a defined point in time (day, month, or year) (Eq. 1, calculation for measuring prevalence):
We selected 68 articles published between 2013 and 2022 that reported cases of ranavirus infections in anurans, confirmed by laboratory techniques. The reports came from countries in all continents, except Antarctica; of these, most (39.7 %) described occurrences in the United States. Consequently, the number of cases recorded in North America was higher than in other continents (33 records or 48.5 % of publications). In contrast to North America, only a few cases have been recorded in continents such as Oceania (1 record) (Wynne, 2020) and Africa (2 records) (Box et al., 2021; Kolby et al., 2015), highlighting a gap in the knowledge of ranaviruses on these continents.
Several species of anurans have been affected by the infection: 16 families were recorded in the reports, including species threatened with extinction (Table 2). Assessed animals came from various sources: free-living animals, animals bred in captivity (commercial farms and aquariums), and zoological collections. It is worth noting that some articles reported the first case of detection of the pathogen in amphibians, either in a natural environment or in captivity (Park et al., 2021; Vörös et al., 2020; Kwon et al., 2017; Ruggeri et al., 2019) in order to create a collaborative, information-exchanging network, demonstrating the increased efforts to identify the cause behind the outbreaks of this emerging disease.
Table 2List of anurans grouped by families and species found in the 68 records selected.
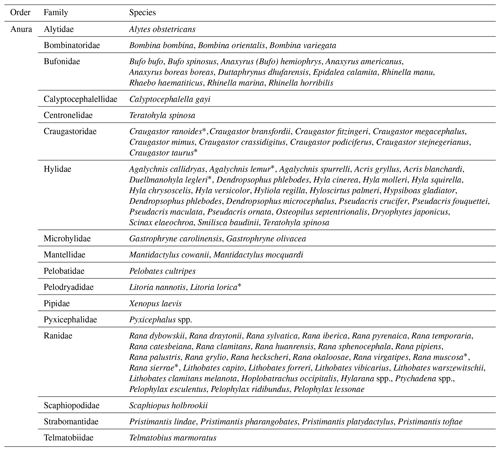
* Species classified as Vulnerable (VU), Endangered (EN), or Critically Endangered (CR) according to the IUCN Red List.
Moreover, some of the studies reported the occurrence of ranavirus infection in species included in the IUCN Red List categories. The concerned species are Craugastor ranoides (Critically Endangered), C. taurus (Endangered), Agalychnis lemur (Critically Endangered), Duellmanohyla legleri (Endangered), Litoria lorica (Critically Endangered), Rana muscosa (Endangered), and R. sierrae (Vulnerable). The assessment considers various factors to define the conservation status of species of different taxa (IUCN, 2023).
Of the 68 articles analyzed, 27 (39.7 %) identified the viral species involved, with Frog virus 3 (FV3) being reported in most cases (13 records). The spatial distribution of ranavirus species in different countries, according to the studies we analyzed, is shown in Fig. 2. Ranaviruses are widespread globally, aided by the international animal trade, whether for the pet market or for food. Studies showed the presence of exported animals with the infection (Kolby et al., 2014), which facilitate the spread of the virus across borders.
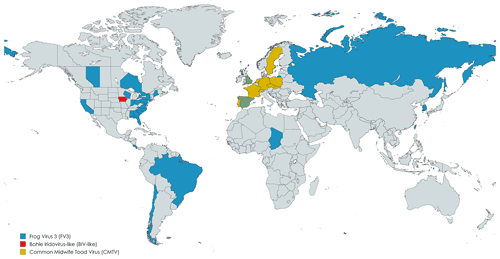
Figure 2Geographical distribution of the ranavirus species and isolates identified in the records. Publisher's remark: please note that the above figure contains disputed territories.
We present the prevalence values found in the articles evaluated, along with each country in which the studies were performed (Table 3). In some cases, it was necessary to calculate the prevalence, as this parameter was not indicated. A new calculation was performed considering only anuran amphibians in articles that presented data for other orders of amphibians. There is a wide variation in prevalence among species and sites, with values reaching 100 % in cases wherein outbreaks with high mortality have been reported (Wheelwright et al., 2014; Miaud et al., 2016; Kwon et al., 2017; Hartmann et al., 2022).
The field of ranavirus studies has seen significant expansion over the past few years. The articles in our study maintain a relative consistency of publications per year (Fig. 3); however, it must be noted that these data represent just a fraction of the extensive volume of publications on this topic. The Global Ranavirus Reporting System is a database created by well-known researchers in the study of ranavirus, and the existing dataset reveals an exponential rise in publications from 2010 onwards (Brunner et al., 2021). On the other hand, the count of scientific reports is not equal to the number of notifications transmitted to WOAH. Black et al. (2017) made such a remark regarding this underreporting, which is reflected in the disparity between articles and official reports. This underscores the need not only for ongoing research but also for informing animal health authorities of cases of ranavirosis.
The decline in endangered species is a matter of significant concern. It is estimated that, since the 1960s, around 200 species of frog have become extinct and that, in the next century, hundreds more may follow a similar path (Alroy, 2015). Many of them are located in biodiversity hotspots, biomes that contain great biological diversity and face a high degree of threat (Mittermeier et al., 2004). Thus, the preservation of ecosystems is of crucial importance for the maintenance of endemic species, both animal and plant.
In addition, invasive species intensify this process: as well as competing for food resources and preying on native species, they have a role as pathogen carriers (Blackburn et al., 2014), serving as a source of infection and acting as conduits in the dynamics of agent transmission. Some articles cited invasive species, such as Eleutherodactylus planirostris (Rivera et al., 2019) and Lithobates catesbeianus (Ruggeri et al., 2019), currently named Aquarana catesbeiana (Jorgewich-Cohen et al., 2022). In one of the articles reviewed, E. planirostris had a higher prevalence and intensity of infection than the other native species evaluated, highlighting the amplifying role that invaders play in disseminating ranaviruses as reservoir hosts (Rivera et al., 2019).
The American bullfrog (A. catesbeiana) is a species widely raised in production for human consumption. This anuran has been introduced into several countries, as various farming sites have been built to breed them on a commercial scale (Ribeiro and Toledo, 2022). However, reports of animals escaping or being abandoned in the wild after the closure of facilities are not uncommon, and the species, which is highly adaptable, has managed to thrive outdoors. In several countries, such as Argentina (Akmentins and Cardozo, 2010), Uruguay (Laufer et al., 2008), and Brazil (Both et al., 2011), feral populations of the bullfrog have been recorded. American bullfrogs have demonstrated resistance to Ranavirus infection (Hoverman et al., 2011) and can carry subclinical levels of the virus (Brunner et al., 2019); this suggests they could impact community transmission dynamics, also acting as reservoirs for the virus.
In addition to intra-class interaction, ranaviruses can be transmitted through inter-class contact since the pathogen can infect different host classes. Studies have reported the occurrence of ranavirus infection in sympatric amphibians and chelonians (Currylow et al., 2014), as well as in anurans and urodeles that share the same territory in a prey–predator relationship (Rothermel et al., 2016). This dynamic favors the transmission of the agent, increasing the likelihood of infection. When considering an environment with great biodiversity, other mobile taxa, such as birds and reptiles, can carry the virus as carriers or reservoirs, contributing to spreading the pathogen in the environment (Tornabene et al., 2018).
Some of the studies we evaluated also highlighted the increased impact of co-infection with other emerging pathogens, including the chytrid fungus Batrachochytrium dendrobatidis (Longcore et al., 1999); this zoospore-forming fungus can infiltrate the host's epidermis, causing a disturbance in its osmoregulatory function, which can result in death (Sewell et al., 2021). One hypothesis to be confirmed is that infection by one of these pathogens may favor subsequent infection by the other (Warne et al., 2016). Seven studies reported animals co-infected with the two pathogens (Whitfield et al., 2013; Reshetnikov et al., 2014; Warne et al., 2016; Olori et al., 2018; Talbott et al., 2018; Watters et al., 2018; Julian et al., 2019).
Co-infection with these pathogens, combined with factors such as climate change, exacerbates the impacts caused by these agents, increasing disease severity and triggering new outbreaks (Thumsová et al., 2022). Hall et al. (2018) observed a relationship between higher ranavirus mortality and higher water temperatures. Temperature is a major factor in the behavior, reproduction, metabolism, and immune function of amphibians (Blaustein et al., 2010), so high temperatures interfere with the full functioning of their bodies, making them susceptible to infection.
The environment is also a key factor in the occurrence of ranavirus infections. Studies showed that certain environmental aspects can increase the predisposition of animals to infection. Elements such as the season or environment-linked aspects (e.g., distance from the nearest lake with a ranavirus infection) can be used to predict infections (Tornabene et al., 2018). The deleterious consequences of human action for the environment also impact amphibians, making them susceptible to infection; for example, ammonia from livestock farming and fertilizers used in agriculture is extremely toxic to aquatic animals and impairs their development and interferes with their immune function, which renders them more vulnerable to the virus (Talbott et al., 2018). A higher severity of infections was also associated with environments contaminated with wastewater that led to an increase in salinity in wetlands (Hall et al., 2020).
Regarding diagnosis, all studies used polymerase chain reaction (PCR) as the primary test to confirm infection; however, other techniques, such as viral isolation (Miaud et al., 2016; Julian et al., 2019), histopathology (Landsberg et al., 2013; Rothermel et al., 2016; Sluijs et al., 2016; Eustace et al., 2018; Hsieh et al., 2021), immunohistochemistry (Forzán et al., 2019), in situ hybridization, and electron microscopy (Hsieh et al., 2021), were also used. Of all the methods used, PCR is the technique recommended by the World Organisation for Animal Health (WOAH) due to its availability, utility, and diagnostic sensitivity and specificity (World Organisation for Animal Health, 2021). The use of samples from the environment to recover environmental DNA (eDNA) is a growing trend, and it was used in three articles retrieved in our search. This method not only eliminates the need to handle and/or euthanize animals but also provides quantitative data on viral elimination in the environment during outbreaks, and it has provided satisfactory results (Hall et al., 2018; Miaud et al., 2019; Tornabene et al., 2021).
Despite the numerous studies reporting ranavirus infections, there is limited understanding of the specific species and strains involved. Among the studies evaluated, the most prevalent species was FV3, followed by CMTV. One study only identified the Bohle iridovirus (BIV) in animals housed in an aquarium with anuran species from various regions, which makes it difficult to identify where the virus originated (Cheng et al., 2014). BIV is a ranavirus frequently found in Australia (Jerrett et al., 2015), and its ability to infect is not limited to amphibians, as cases of epizootic outbreaks have already been reported in bony fish and reptiles (Chinchar et al., 2017). A notable study documented the diagnosis and molecular characterization through phylogenetic analysis of a ranavirus strain in Brazil (Oliveira et al., 2020). This strain exhibited a distinct phylogenetic profile compared to other strains identified globally. We emphasize that studies providing molecular data of detected Ranavirus species and strains (i.e., sequencing) are crucial for uncovering the evolutionary history of the virus. Such data can also shed light on genes that may influence the replication cycle and viral pathogenesis.
We would also highlight the lack of studies on ranavirus infections in continents such as Africa and Oceania; although the presence of the virus is known, there is a scarcity of information on the distribution of the pathogen in the territories and on which species are prevalent, as well as on which animal species are susceptible to the virus. We therefore suggest that more studies on ranavirus infection should be carried out in order to increase knowledge of the virus distribution and prevent outbreaks.
In epidemiology, coefficients are essential for measuring events related to the health–disease process. The prevalence coefficient, point prevalence (or simply prevalence), is one of the most commonly used measures of disease frequency. It quantifies the proportion of individuals in a population affected by a disease at a specific time. Wagner (1998) likens this indicator to a photograph, capturing a static moment in time (even if data collection spans days, months, or years). Thus, it is vital to measure the probability of morbid events (Merchán-Hamann et al., 2000) and evaluate the frequency of disease risk factors (Lima et al., 2018). Prevalence studies are helpful when investigating wildlife mortality events; however, assessing the death rate is a difficult task, as it is not always possible to record all the dead animals. For this reason, for diseases of relevance to wildlife, measuring the point prevalence is recommended; this is done by sampling specimens from the target population, whether these animals are alive or dead (Mörner et al., 2002).
Regarding the prevalence data of our study, it is noteworthy that lower values do not necessarily imply a lower occurrence of infection compared to other cases. For instance, Miaud et al. (2016) described a ranavirus outbreak where hundreds of dead animals were observed in three lakes in the southeastern Alps, France. In that study, 21 animals were collected for the diagnostic test, and all tested positive. In contrast, Price et al. (2017) conducted a 25-year retrospective study evaluating the occurrence of infections in individuals from an archive. Although the number of positive animals detected was higher (87), the prevalence was low due to the large number of tested individuals.
Equal importance must be given to studies with negative data. Ten of the studies evaluated showed negative results, in which the pathogen investigated was not detected (Titus and Green, 2013; Richter et al., 2013; Sainsbury et al., 2017; Eustace et al., 2018; Hall et al., 2018; Goodman et al., 2019; Jacinto-Maldonado et al., 2020; Tornabene et al., 2021; Kostanjšek et al., 2021; Strachinis et al., 2022). Negative data are sometimes neglected and rarely published; however, data such as these are valuable for a better understanding of the epidemiology of the disease and of its distribution and infection patterns according to different species and time periods (Stallknecht, 2007). Besides, due to the damage caused by this disease, null results should be valued from a conservation perspective.
Eradicating a disease that circulates among wild animals is quite difficult; therefore, prevention and risk analysis are necessary to avoid the spread of ranaviruses. Sainsbury et al. (2017) described the application of a program for the reintroduction of Pelophylax lessonae for the conservation of this species. In this sense, quarantine and testing of animals to be reintroduced into the wild are essential to ensure that these animals do not introduce pathogens into the receiving environment. If an outbreak is already in progress, the goals are the early detection of the pathogen and preventing its dissemination. One management action aiming to restrict the spread of the disease is the removal of host species, as reported by Martel et al. (2020) in relation to a Batrachochytrium salamandrivorans outbreak in Spain; however, this measure has been highly questioned and should not be carried out when the species in question is at risk of extinction (Bozzuto et al., 2020). For captive animals, control and prevention of the disease are based on preventive management measures (quarantine, sanitization of the facilities), as well as on the elimination of infected animals (Batista et al., 2021). There are currently no vaccines available, although there is ongoing research into the development of immunizers against ranavirus (Chen et al., 2018; Zhang et al., 2012).
The global rise in Ranavirus infections among amphibians, particularly anurans, highlights the urgent need for coordinated efforts in disease surveillance and management. Despite the increasing number of reports, several knowledge gaps remain, especially in regions where infections have not been thoroughly studied. To mitigate the impact on vulnerable species and ecosystems, a multi-faceted approach to surveillance, early detection, control, and post-outbreak analysis is essential. The following key points outline the critical actions required to advance our understanding of ranavirosis:
- -
Exploratory research in new locations. While reports of Ranavirus are becoming more frequent, there is a pressing need for comprehensive epidemiological studies in regions where the infection status remains unknown.
- -
Molecular characterization. Accurate identification of Ranavirus species through molecular characterization is crucial for understanding viral diversity, guiding control measures, and predicting potential future outbreaks.
- -
Surveillance for conservation. Implementing targeted surveillance programs is vital, particularly for endangered species, to monitor emerging infectious diseases and ensure timely reporting to both national and international animal health authorities.
- -
Post-outbreak monitoring. Post-outbreak strategies, such as serological testing, are critical to determine the extent of infection and evaluate the health status of surviving populations.
The authors are open to sharing any information and data about the research upon contact and request.
JWPJ and DdSR: conceptualization; JWPJ: methodology; RdCCM, GJBdM, and RLMdS: validation; DdSR: formal analysis, investigation, data curation, and writing (original draft preparation); JWPJ, RdCCM, GJBdM, and RLMdS: writing (review and editing); JWPJ, RdCCM, GJBdM, and RLMdS: visualization; JWPJ and RdCCM: supervision. All authors have read and agreed to the published version of the article.
The contact author has declared that none of the authors has any competing interests.
Publisher’s note: Copernicus Publications remains neutral with regard to jurisdictional claims made in the text, published maps, institutional affiliations, or any other geographical representation in this paper. While Copernicus Publications makes every effort to include appropriate place names, the final responsibility lies with the authors.
This paper was edited by Ricardo Rocha and reviewed by two anonymous referees.
Akmentins, M. S. and Cardozo, D. E.: American bullfrog Lithobates catesbeianus (Shaw, 1802) invasion in Argentina, Biol. Invasions, 12, 735–737, https://doi.org/10.1007/s10530-009-9515-3, 2010.
Alroy, J.: Current extinction rates of reptiles and amphibians, P. Natl. Acad. Sci. USA, 112, 13003–13008, https://doi.org/10.1073/pnas.1508681112, 2015.
Bates, K. A., Friesen, J., Loyau, A., Butler, H., Vredenburg, V. T., Laufer, J., Chatzinotas, A., and Schmeller, D. S.: Environmental and Anthropogenic Factors Shape the Skin Bacterial Communities of a Semi-Arid Amphibian Species, Microb. Ecol., 86, 1393–1404, https://doi.org/10.1007/s00248-022-02130-5, 2023.
Batista, C. L. M., de Vieira, A. N. V. B., and Lopes, J. C. O.: Frog Virology: Biosafety in an Experimental Farm, in: Current Perspectives on Viral Disease Outbreaks-Epidemiology, Detection and Control, IntechOpen, 1–13, https://doi.org/10.5772/intechopen.96605, 2021.
Bielby, J., Price, S. J., Monsalve-Carcaño, C., and Bosch, J.: Host contribution to parasite persistence is consistent between parasites and over time, but varies spatially, Ecol. Appl., 31, e02256, https://doi.org/10.1002/eap.2256, 2021.
Bienentreu, J.-F., Schock, D. M., Greer, A. L., and Lesbarrères, D.: Ranavirus Amplification in Low-Diversity Amphibian Communities, Frontiers in Veterinary Science, 9, 755426, https://doi.org/10.3389/fvets.2022.755426, 2022.
Black, Y., Meredith, A., and Price, S. J.: Detection and reporting of ranavirus in amphibians: evaluation of the roles of the World Organisation for Animal Health and the published literature, J. Wildlife Dis., 53, 509–520, https://doi.org/10.7589/2016-08-176, 2017.
Blackburn, T. M., Essl F., Evans T., Hulme P. E., Jeschke J. M., Kühn I., Kumschick, S., Markova, Z., Mrugała, A., Nentwig W., Pergl, J., Pyšek, P., Rabitsch, W., Ricciardi, A., Richardson, D. M., Sendek A., Vilà, M., Wilson J. R. U., Winter M., Genovesi, P., and Bacher S.: A Unified Classification of Alien Species Based on the Magnitude of their Environmental Impacts, PLoS Biol., 12, e1001850, https://doi.org/10.1371/journal.pbio.1001850, 2014.
Blaustein, A. R., Walls, S. C., Bancroft, B. A., Lawler, J. J., Searle, C. L., and Gervasi, S. S.: Direct and indirect effects of climate change on amphibian populations, Diversity, 2, 281–313, https://doi.org/10.3390/d2020281, 2010.
Bosch, J., Mora-Cabello de Alba, A., Marquínez, S., Price, S. J., Thumsová, B., and Bielby, J.: Long-Term Monitoring of Amphibian Populations of a National Park in Northern Spain Reveals Negative Persisting Effects of Ranavirus, but not Batrachochytrium dendrobatidis, Frontiers in Veterinary Science, 8, 645491, https://doi.org/10.3389/fvets.2021.645491, 2021.
Both, C., Lingnau, R., Santos Jr., A., Madalozzo, B., Lima, L. P., and Grant, T.: Widespread Occurrence of the American Bullfrog, Lithobates catesbeianus (Shaw, 1802) (Anura: Ranidae), in Brazil, S. Am. J. Herpetol., 6, 127–134, https://doi.org/10.2994/057.006.0203, 2011.
Box, E. K., Cleveland, C. A., Subramaniam, K., Waltzek, T. B., and Yabsley, M. J.: Molecular Confirmation of Ranavirus Infection in Amphibians from Chad, Africa, Frontiers in Veterinary Science, 8, 733939, https://doi.org/10.3389/fvets.2021.733939, 2021.
Bozzuto, C., Schmidt, B. R., and Canessa, S.: Active responses to outbreaks of infectious wildlife diseases: objectives, strategies and constraints determine feasibility and success, P. Roy. Soc. B-Biol. Sci., 287, 20202475, https://doi.org/10.1098/rspb.2020.2475, 2020.
Brenes, R., Gray, M. J., Waltzek, T. B., Wilkes, R. P., and Miller, D. L.: Transmission of Ranavirus between Ectothermic Vertebrate Hosts, PLoS ONE, 9, e92476, https://doi.org/10.1371/journal.pone.0092476, 2014.
Brunner, J., Olson, A., Rice, J., Meiners, S., Le Sage, M., Cundiff, J., Goldberg, C., and Pessier, A.: Ranavirus infection dynamics and shedding in American bullfrogs: consequences for spread and detection in trade, Dis. Aquat. Organ., 135, 135–150, https://doi.org/10.3354/dao03387, 2019.
Brunner, J. L., Olson, D. H., Gray, M. J., Miller, D. L., and Duffus, A. L. J.: Global patterns of ranavirus detections, FACETS, 6, 912–924, https://doi.org/10.1139/facets-2020-0013, 2021.
Bryan, L., Baldwin, C., Gray, M., and Miller, D.: Efficacy of select disinfectants at inactivating Ranavirus, Dis. Aquat. Organ., 84, 89–94, https://doi.org/10.3354/dao02036, 2009.
Chen, Z. Y., Li, T., Gao, X. C., Wanf, C. F., and Zhang, Q. Y.: Protective immunity induced by DNA vaccination against ranavirus infection in Chinese giant salamander Andrias davidianus, Viruses, 10, 52, https://doi.org/10.3390/v10020052, 2018.
Cheng, K., Jones, M. E., Jancovich, J. K., Burchell, J., Schrenzel, M. D., Reavill, D. R., Imai, D. M., Urban, A., Kirkendall, M., Woods, L. W., Chinchar, V. G., and Pessier, A. P.: Isolation of a Bohle-like iridovirus from boreal toads housed within a cosmopolitan aquarium collection, Dis. Aquat. Organ., 111, 139–152, https://doi.org/10.3354/dao02770, 2014.
Chinchar, V. G., Hick, P., Ince, I. A., Jancovich, J. K., Marschang, R., Qin, Q., Subramaniam, K., Waltzek, T. B., Whittington, R., Williams, T., and Zhang, Q. Y.: ICTV virus taxonomy profile: Iridoviridae, J. Gen. Virol., 98, 890–891, https://doi.org/10.1099/jgv.0.000818, 2017.
Crespi, E. J., Rissler, L. J., Mattheus, N. M., Engbrecht, K., Duncan, S. I., Seaborn, T., Hall, E. M., Peterson, J. D., and Brunner, J. L.: Geophysiology of Wood Frogs: Landscape Patterns of Prevalence of Disease and Circulating Hormone Concentrations across the Eastern Range, Integr. Comp. Biol., 55, 602–617, https://doi.org/10.1093/icb/icv096, 2015.
Cunningham, A. A., Hyatt, A. D., Russell, P., and Bennett, P. M.: Experimental transmission of a ranavirus disease of common toads (Bufo bufo) to common frogs (Rana temporaria), Epidemiol. Infect., 135, 1213–1216, https://doi.org/10.1017/S0950268807007935, 2007.
Currylow, A. F., Johnson, A. J., and Williams, R. N.: Evidence of ranavirus infections among sympatric larval amphibians and box turtles, J. Herpetol., 48, 117–121, https://doi.org/10.1670/12-235, 2014.
D'Aoust-Messier, A., Echaubard, P., Billy, V., and Lesbarrères, D.: Amphibian pathogens at northern latitudes: presence of chytrid fungus and ranavirus in northeastern Canada, Dis. Aquat. Organ., 113, 149–155, https://doi.org/10.3354/dao02837, 2015.
Daszak, P., Cunningham, A. A., and Hyatt, A. D.: Infectious disease and amphibian population declines, Divers. Distrib., 9, 141–150, https://doi.org/10.1046/j.1472-4642.2003.00016.x, 2003.
Davis, D. R., Farkas, J. K., Kruisselbrink, T. R., Watters, J. L., Ellsworth, E. D., Kerby, J. L., and Siler, C. D.: Prevalence and distribution of ranavirus in amphibians from southeastern Oklahoma, USA, Herpetol. Conserv. Bio., 14, 360–369, 2019.
De, E., Leite, L., Santi, M., and Corrêa, A. C.: Mata Atlântica: a sobrevivência dos anfíbios/Atlantic Forest: the survival of the amphibians, FESPPR Publica, 2, 1–12, https://doi.org/10.1007/978-3-319-13755-1_2, 2018.
Duffus, A. L. J., Nichols, R. A., and Garner, T. W. J.: Detection of a Frog Virus 3-like Ranavirus in Native and Introduced Amphibians in the United Kingdom in 2007 and 2008, Herpetological Review, 45, 608–610, 2014.
Duffus, A. L. J., Waltzek, T. B., Stöhr, A. C., Allender, A. M., Gotesman, M., Whittington, R. J., Hick, P., Hines, M. K., and Marschang, R. E.: Distribution and Host Range of Ranaviruses, in: Ranaviruses Lethal Pathogens of Ectothermic Vertebrates, edited by: Gray, M. J. and Chinchar V. G., Springer, 9–57, 2015.
Earl, J. E., Chaney, J. C., Sutton, W. B., Lillard, C. E., Kouba, A. J., Langhorne, C., Krebs, J., Wilkes, R. P., Hill, R. D., Miller, D. L., and Gray, M. J.: Ranavirus could facilitate local extinction of rare amphibian species, Oecologia, 182, 611–623, https://doi.org/10.1007/s00442-016-3682-6, 2016.
Eustace, R., Wack, A., Mangus, L., and Bronson, E.: Causes of mortality in captive Panamanian Golden frogs (Atelopus zeteki) at the Maryland ZOO in Baltimore, 2001–2013, J. Zoo Wildlife Med., 49, 324–334, https://doi.org/10.1638/2016-0250.1, 2018.
Ford, C. E., Brookes, L. M., Skelly, E., Sergeant, C., Jordine, T., Balloux, F., Nichols, R. A., and Garner, T. W. J.: Non-Lethal Detection of Frog Virus 3-Like (RUK13) and Common Midwife Toad Virus-Like (PDE18) Ranaviruses in Two UK-Native Amphibian Species, Viruses, 14, 2635, https://doi.org/10.3390/v14122635, 2022.
Forzán, M. J. and Wood, J.: Low detection of ranavirus DNA in wild postmetamorphic green frogs, Rana (Lithobates) clamitans, despite previous or concurrent tadpole mortality, J. Wildlife Dis., 49, 879–886, https://doi.org/10.7589/2013-03-051, 2013.
Forzán, M. J., Jones, K. M., Vanderstichel, R. V., Wood, J., Kibenge, F. S. B., Kuiken, T., Wirth, W., Ariel, E., and Daoust, P.-Y.: Clinical signs, pathology and dose-dependent survival of adult wood frogs, Rana sylvatica, inoculated orally with frog virus 3 Ranavirus sp., Iridoviridae, J. Gen. Virol., 96, 1138–1149, https://doi.org/10.1099/vir.0.000043, 2015.
Forzán, M. J., Jones, K. M., Ariel, E., Whittington, R. J., Wood, J., Markham, R. J. F., and Daoust, P. Y.: Pathogenesis of Frog Virus 3 (Ranavirus, Iridoviridae) Infection in Wood Frogs (Rana sylvatica), Vet. Pathol., 54, 531–548, https://doi.org/10.1177/0300985816684929, 2017.
Forzán, M., Bienentreu, J., Schock, D., and Lesbarrères, D.: Multi-tool diagnosis of an outbreak of ranavirosis in amphibian tadpoles in the Canadian boreal forest, Dis. Aquat. Organ., 135, 33–41, https://doi.org/10.3354/dao03369, 2019.
Frost, D. R.: Amphibian Species of the World: an Online Reference, Version 6.2, American Museum of Natural History, New York, USA, https://amphibiansoftheworld.amnh.org/index.php (last access: 28 August 2024), 2024.
Galt, N., Atkinson, M., Glorioso, B. M., Hardin Waddle, J., Litton, M., and Savage, A.: Widespread ranavirus and perkinsea infections in Cuban treefrogs (Osteopilus septentrionalis) invading New Orleans, USA, Herpetol. Conserv. Biol., 16, 17–29, 2021.
Goodman, R. M., Tyler, J. A., Reinartz, D. M., and Wright, A. N.: Survey of Ranavirus and Batrachochytrium dendrobatidis in Introduced Frogs in Hawaii, USA, J. Wildlife Dis., 55, 668, https://doi.org/10.7589/2018-05-137, 2019.
Grant, S., Bienentreu, J., Vilaça, S., Brunetti, C., Lesbarrères, D., Murray, D., and Kyle, C. J.: Low intraspecific variation of Frog virus 3 with evidence for novel FV3-like isolates in central and northwestern Canada, Dis. Aquat. Organ., 134, 1–13 https://doi.org/10.3354/dao03354, 2019.
Gray, M., Miller, D., and Hoverman, J.: Reliability of non-lethal surveillance methods for detecting ranavirus infection, Dis. Aquat. Organ., 99, 1–6, https://doi.org/10.3354/dao02436, 2012.
Gray, M. J. and Miller, D. L.: The Rise of Ranavirus, The Wildlife Society, 51–55, 2013.
Hall, E. M., Goldberg, C. S., Brunner, J. L., and Crespi, E. J.: Seasonal dynamics and potential drivers of ranavirus epidemics in wood frog populations, Oecologia, 188, 1253–1262, https://doi.org/10.1007/s00442-018-4274-4, 2018.
Hall, E. M., Brunner, J. L., Hutzenbiler, B., and Crespi, E. J.: Salinity stress increases the severity of ranavirus epidemics in amphibian populations, P. Roy. Soc. B-Biol. Sci., 287, 20200062, https://doi.org/10.1098/rspb.2020.0062, 2020.
Harp, E. M. and Petranka, J. W.: Ranavirus in wood frogs (Rana sylvatica): potential sources of transmission within and between ponds, J. Wildlife Dis., 42, 307–318, https://doi.org/10.7589/0090-3558-42.2.307, 2006.
Hartmann, A. M., Maddox, M. L., Ossiboff, R. J., and Longo, A. V.: Sustained ranavirus outbreak causes mass mortality and morbidity of imperiled amphibians in Florida, EcoHealth, 19, 8–14, https://doi.org/10.1007/s10393-021-01572-6, 2022.
Horner, A. A., Hoffman, E. A., Tye, M. R., Hether, T. D., and Savage, A. E.: Cryptic chytridiomycosis linked to climate and genetic variation in amphibian populations of the southeastern United States, PLoS ONE, 12, e0175843, https://doi.org/10.1371/journal.pone.0175843, 2017.
Hoverman, J. T., Gray, M. J., Haislip, N. A., and Miller, D. L.: Phylogeny, life history, and ecology contribute to differences in amphibian susceptibility to ranaviruses, EcoHealth, 8, 301–319, https://doi.org/10.1007/s10393-011-0717-7, 2011.
Hsieh, C.-Y., Rairat, T., and Chou, C.-C.: Detection of Ranavirus by PCR and in situ hybridization in the American bullfrog (Rana catesbeiana) in Taiwan, Aquaculture, 543, 736955, https://doi.org/10.1016/j.aquaculture.2021.736955, 2021.
IUCN (International Union for Conservation of Nature): The IUCN Red list of threatened species, Version 2023-1, https://www.iucnredlist.org/, last access: 13 November 2023.
Jacinto-Maldonado, M., García-Peña, G. E., PParedes-León, R., Saucedo, B., Sarmiento-Silva, R. E., García, A., Martínez-Gómez, D., Ojeda, M., Del Callejo, E., and Suzán, G.: Chiggers (Acariformes: Trombiculoidea) do not increase rates of infection by Batrachochytrium dendrobatidis fungus in the endemic Dwarf Mexican Treefrog Tlalocohyla smithii (Anura: Hylidae), International Journal for Parasitology: Parasites and Wildlife, 11, 163–173, https://doi.org/10.1016/j.ijppaw.2019.12.005, 2020.
Jancovich, J. K., Chinchar, V. G., Hyatt, A., Miyazaki, T., Williams, T., and Zhang, Q. Y.: Family Iridoviridae, in: Virus Taxonomy: Ninth Report of the International Committee on Taxonomy of Viruses, edited by: King, A. M. S., Adams, M. J., Carstens, E. B., and Lefkowitz, E. J., Elsevier Academic Press, 193–210, ISBN 978-0-12-384684-6, 2012.
Jerrett, I. V., Whittington, R. J., and Weir, R. P.: Pathology of a Bohle-like virus infection in two Australian frog species (Litoria splendida and Litoria caerulea), J. Comp. Pathol., 152, 248–259, https://doi.org/10.1016/j.jcpa.2014.12.007, 2015.
Jorgewich-Cohen, G., Toledo, L. F., and Grant, T.: Genetic structure of American bullfrog populations in Brazil, Sci. Rep., 12, 9927, https://doi.org/10.1038/s41598-022-13870-2, 2022.
Julian, J. T., Brooks, R. P., Glenney, G. W., and Coll, J. A.: State-wide Survey of amphibian pathogens in green frog (Lithobates clamitans melanota) reveals high Chytrid infection intensities in constructed wetlands, Herpetol. Conserv. Bio., 14, 199–211, 2019.
Karwacki, E. E., Martin, K. R., and Savage, A. E.: One hundred years of infection with three global pathogens in frog populations of Florida, USA, Biol. Conserv., 257, 1–12, https://doi.org/10.1016/j.biocon.2021.109088, 2021.
Kolby, J. E., Smith, K. M., Berger, L., Karesh, W. B., Preston, A., Pessier, A. P., and Skerratt, L. F.: First Evidence of Amphibian Chytrid Fungus (Batrachochytrium dendrobatidis) and Ranavirus in Hong Kong Amphibian Trade, PLoS ONE, 9, e90750, https://doi.org/10.1371/journal.pone.0090750, 2014.
Kolby, J. E., Smith, K. M., Ramirez, S. D., Rabemananjara, F., Pessier, A. P., Brunner, J. L., Goldberg, C. S., Berger, L., and Skerratt, L. F.: Rapid Response to Evaluate the Presence of Amphibian Chytrid Fungus (Batrachochytrium dendrobatidis) and Ranavirus in Wild Amphibian Populations in Madagascar, PLoS ONE, 10, e0125330, https://doi.org/10.1371/journal.pone.0125330, 2015.
Kostanjšek, R., Turk, M., Vek, M., Gutiérrez-Aguirre, I., and Cimerman, N. G.: First screening for Batrachochytrium dendrobatidis, B. salamandrivorans and Ranavirus infections in wild and captive amphibians in Slovenia, Salamandra, 57, 162–166, 2021.
Kraus, F.: Impacts from Invasive Reptiles and Amphibians, Annu. Rev. Ecol. Evol. S., 46, 75–97, https://doi.org/10.1146/annurev-ecolsys-112414-054450, 2015.
Kwon, S., Park, J., Choi, W.-J., Koo, K.-S., Lee, J.-G., and Park, D.: First case of ranavirus-associated mass mortality in a natural population of the Huanren frog (Rana huanrenensis) tadpoles in South Korea, Anim. Cells Syst., 21, 358–364, https://doi.org/10.1080/19768354.2017.1376706, 2017.
Landsberg, J., Kiryu, Y., Tabuchi, M., Waltzek, T., Enge, K., Reintjes-Tolen, S., Preston, A., and Pessier, A.: Co-infection by alveolate parasites and frog virus 3-like ranavirus during an amphibian larval mortality event in Florida, USA, Dis. Aquat. Organ., 105, 89–99, https://doi.org/10.3354/dao02625, 2013.
Latney, L. V. and Klaphake, E.: Selected Emerging Diseases of Amphibia, Vet. Clin. North Am. Exot. Anim. Pract., 16, 283–301, https://doi.org/10.1016/j.cvex.2013.01.005, 2013.
Laufer, G., Canavero, A., Núñez, D., and Maneyro, R.: Bullfrog (Lithobates catesbeianus) invasion in Uruguay, Biol. Invasions, 10, 1183–1189, https://doi.org/10.1007/s10530-007-9178-x, 2008.
Lima, J. R. C., Pordeu, J. M. C., and Rouquayrol, M. Z.: Medida da Saúde Coletiva, in: Epidemiologia e Saúde, edited by: Rouquayrol, M. Z. and Silva, M. G. C., 8th edn., Medbook, 108–200, 2018.
Love, C., Winzeler, M., Beasley, R., Scott, D., Nunziata, S., and Lance, S.: Patterns of amphibian infection prevalence across wetlands on the Savannah River Site, South Carolina, USA, Dis. Aquat. Organ., 121, 1–14, https://doi.org/10.3354/dao03039, 2016.
Longcore, J. E., Pessier, A. P., and Nichols, D. K.: Batrachochytrium dendrobatidis gen. et sp. nov., a chytrid pathogenic to amphibians, Mycologia, 91, 219–227, 1999.
Maclachlan, N. J. and Dubovi, E. J. (Eds.): Asfarviridae and Iridoviridae. in: Fenner's Veterinary Virology, 5th edn., Elsevier, 175–188, ISBN: 9780128011706, 2016.
Martel, A., Vila-Escale, M., Fernández-Giberteau, D., Martinez-Silvestre, A., Canessa, S., Praet, S. V., Pannon, P., Chiers, K., Ferran, A., Kelly, M., Picart, M., Piulats, D., Li, Z., Pagone, V., Pérez-Sorribes, L., Molina, C., Tarragó-Guarro, A., Velarde-Nieto, R., Carbonell, F., Obon, E., Martinéz-Martinéz, D., Guinart, D., Casanovas, R., Carranza, S., and Pasmans, F.: Integral chain management of wildlife diseases, Conserv. Lett., 13, e12707, https://doi.org/10.1111/conl.12707, 2020.
Merchán-Hamann, E., Tauil, P. L., and Costa, M. P.: Terminologia das medidas e indicadores em epidemiologia: Subsídios para uma possível padronização da nomenclatura, Inf. Epidemiol. Sus, 9, 276–284, https://doi.org/10.5123/S0104-16732000000400006, 2000.
Miaud, C., Pozet, F., Gaudin, N. C. G., Martel, A., Pasmans, F., and Labrut, S.: Ranavirus causes mass die-offs of alpine amphibians in the southwestern Alps, France, J. Wildlife Dis., 52, 242–252, https://doi.org/10.7589/2015-05-113, 2016.
Miaud, C., Arnal, V., Poulain, M., Valentini, A., and Dejean, T.: eDNA Increases the Detectability of Ranavirus Infection in an Alpine Amphibian Population, Viruses, 11, 526, https://doi.org/10.3390/v11060526, 2019.
Miller, D. L., Rajeev, S., Gray, M. J., and Baldwin, C. A.: Frog virus 3 infection, cultured American bullfrogs, Emerg. Infect. Dis., 13, 342–343, https://doi.org/10.3201/eid1302.061073, 2007.
Mittermeier, R. A., Gil, P. R., Hoffmann, M., Pilgrim, J., Brooks, J., Mittermeier, C. G., Lamouroux, J., and Fonseca, G. A. B.: Hotspots Revisited: Earth's Biologically Richest and Most Endangered Terrestrial Ecoregions, Cemex, Washington, DC, 390 pp., ISBN 968-6397-77-9, 2004.
Morales, H. D., Abramowitz, L., Gertz, J., Sowa, J., Vogel, A., and Robert, J.: Innate Immune Responses and Permissiveness to Ranavirus Infection of Peritoneal Leukocytes in the Frog Xenopus laevis, J. Virol., 84, 4912–4922, https://doi.org/10.1128/jvi.02486-09, 2010.
Mörner, T., Obendorf, D. L., Artois, M., and Woodford, M. H.: Surveillance and monitoring of wildlife diseases, Rev. Sci. Tech. OIE, 21, 67–76, https://doi.org/10.20506/rst.21.1.1321, 2002.
Mosher, B. A., Brand, A. B., Wiewel, A. N., Miller, D. A., Gray, M. J., Miller, D. L., and Grant, E. H. C.: Estimating occurrence, prevalence, and detection of amphibian pathogens: insights from occupancy models, J. Wildlife Dis., 55, 563–575, https://doi.org/10.7589/2018-02-042, 2019.
Munro, J., Bayley, A. E., McPherson, N. J., and Feist, S. W.: Survival of Frog Virus 3 in Freshwater and Sediment from an English Lake, J. Wildlife Dis., 52, 138–142, https://doi.org/10.7589/2015-02-033, 2016.
Nazir, J., Spengler, M., and Marschang, R.: Environmental persistence of amphibian and reptilian ranaviruses, Dis. Aquat. Organ., 98, 177–184, https://doi.org/10.3354/dao02443, 2012.
Oliveira, C. R., Alfaia, S. R., Ikari, F. L., Tavares, L. S., Sousa, R. L. M. de, Harakava, R., and Ferreira, C. M.: Detection and molecular characterization of Frog virus 3 in bullfrogs from frog farms in Brazil, Aquaculture, 516, 734575, https://doi.org/10.1016/j.aquaculture.2019.734575, 2020.
Olori, J., Netzband, R., McKean, N., Lowery, J., Parsons, K., and Windstam, S.: Multi-year dynamics of ranavirus, chytridiomycosis, and co-infections in a temperate host assemblage of amphibians, Dis. Aquat. Organis., 130, 187–197, https://doi.org/10.3354/dao03260, 2018.
Palomar, G., Jakóbik, J., Bosch, J., Kolenda, K., Kaczmarski, M., Jośko, P., Roces-Díaz, J., Stachyra, P., Thumsová, B., Zieliński, P., and Pabijan, M.: Emerging infectious diseases of amphibians in Poland: distribution and environmental drivers, Dis. Aquat. Organ., 147, 1–12, https://doi.org/10.3354/dao03631, 2021.
Park, J., Grajal-Puche, A., Roh, N.-H., Park, I.-K., Ra, N.-Y., and Park, D.: First detection of ranavirus in a wild population of Dybowski's brown frog (Rana dybowskii) in South Korea, Journal of Ecology and Environment, 45, 2, https://doi.org/10.1186/s41610-020-00179-2, 2021.
Picco, A. M. and Collins, J. P.: Amphibian Commerce as a Likely Source of Pathogen Pollution, Conserv. Biol., 22, 1582–1589, https://doi.org/10.1111/j.1523-1739.2008.01025.x, 2008.
Price, S. J., Garner, T. W. J., Nichols, R. A., Balloux, F., Ayres, C., Mora-Cabello de Alba, A., and Bosch, J.: Collapse of Amphibian Communities Due to an Introduced Ranavirus, Curr. Biol., 24, 2586–2591, https://doi.org/10.1016/j.cub.2014.09.028, 2014.
Price, S. J., Wadia, A., Wright, O. N., Leung, W. T. M., Cunningham, A. A., and Lawson, B.: Screening of a long-term sample set reveals two Ranavirus lineages in British herpetofauna, PLoS ONE, 12, e0184768, https://doi.org/10.1371/journal.pone.0184768, 2017.
Puschendorf, R., Wallace, M., Chavarría, M. M., Crawford, A. J., Wynne, F., Knight, M., Janzen, D. H., Hallwachs, W., Palmer, C. V., and Price, S. J.: Cryptic diversity and ranavirus infection of a critically endangered Neotropical frog before and after population collapse, Anim. Conserv., 22, 515–524, https://doi.org/10.1111/acv.12498, 2019.
Reshetnikov, A., Chestnut, T., Brunner, J., Charles, K., Nebergall, E., and Olson, D.: Detection of the emerging amphibian pathogens Batrachochytrium dendrobatidis and ranavirus in Russia, Dis. Aquat. Organ., 110, 235–240, https://doi.org/10.3354/dao02757, 2014.
Ribeiro, L. P. and Toledo, L. F.: An overview of the Brazilian frog farming, Aquaculture, 548, 737623, https://doi.org/10.1016/j.aquaculture.2021.737623, 2022.
Richter, S. C., Drayer, A. N., Strong, J. R., and Cross, C. S.: High prevalence of ranavirus infection in permanent constructed wetlands in eastern Kentucky, USA, Herpetological Review, 44, 464–466, 2013.
Rivera, B., Cook, K., Andrews, K., Atkinson, M. S., and Savage, A. E.: Pathogen Dynamics in an Invasive Frog Compared to Native Species, EcoHealth, 16, 222–234, https://doi.org/10.1007/s10393-019-01432-4, 2019.
Roh, N., Park, J., Kim, J., Kwon, H., and Park, D.: Prevalence of Ranavirus Infection in Three Anuran Species across South Korea, Viruses, 14, 1073, https://doi.org/10.3390/v14051073, 2022.
Rosa, G. M., Sabino-Pinto, J., Laurentino, T. G., Martel, A., Pasmans, F., Rebelo, R., Griffiths, R. A., Stöhr, A. C., Marschang, R. E., Price, S. J., Garner, T. W. J., and Bosch, J.: Impact of asynchronous emergence of two lethal pathogens on amphibian assemblages. Sci. Rep., 7, 43260, https://doi.org/10.1038/srep43260, 2017.
Rothermel, B., Miller, D., Travis, E., Gonynor McGuire, J., Jensen, J., and Yabsley, M.: Disease dynamics of red-spotted newts and their anuran prey in a montane pond community, Dis. Aquat. Organ., 118, 113–127, https://doi.org/10.3354/dao02965, 2016.
Ruggeri, J., Ribeiro, L. P., Pontes, M. R., Toffolo, C., Candido, M., Carriero, M. M., Zanella, N., Sousa, R. L. M., and Toledo, L. F.: Discovery of Wild Amphibians Infected with Ranavirus in Brazil, J. Wildlife Dis., 55, 897, https://doi.org/10.7589/2018-09-224, 2019.
Sainsbury, A. W., Yu-Mei, R., Ågren, E., Vaughan-Higgins, R. J., Mcgill, I. S., Molenaar, F., Peniche, G., and Foster, J.: Disease Risk Analysis and Post-Release Health Surveillance for a Reintroduction Programme: The Pool Frog Pelophylax lessonae, Transbound. Emerg. Dis., 64, 1530–1548, https://doi.org/10.1111/tbed.12545, 2017.
Santi, E. de E. L. M. and Corrêa, A. C.: Mata Atlântica: a sobrevivência dos anfíbios/Atlantic Forest: the survival of the amphibians, FESPPR Publica, 2, 1–12, 2018.
Savage, A. E., Muletz-Wolz, C. R., Campbell Grant, E. H., Fleischer, R. C., and Mulder, K. P.: Functional variation at an expressed MHC class IIβ locus associates with Ranavirus infection intensity in larval anuran populations, Immunogenetics, 71, 335–346, https://doi.org/10.1007/s00251-019-01104-1, 2019.
Schock, D. M., Bollinger, T. K., Gregory Chinchar, V., Jancovich, J. K., and Collins, J. P.: Experimental Evidence that Amphibian Ranaviruses Are Multi-Host Pathogens, Copeia, 2008, 133–143, https://doi.org/10.1643/CP-06-134, 2008.
Sewell, T. R., Longcore, J., and Fisher, M. C.: Batrachochytrium dendrobatidis, Trends Parasitol., 37, 933–934, https://doi.org/10.1016/j.pt.2021.04.014, 2021.
Sluijs, A. S. der, van den Broek, J., Kik, M., Martel, A., Janse, J., van Asten, F., Pasmans, F., Gröne, A., and Rijks, J. M.: Monitoring ranavirus-associated mortality in a Dutch heathland in the aftermath of a ranavirus disease outbreak, J. Wildlife. Dis., 52, 817–827, https://doi.org/10.7589/2015-04-104, 2016.
Smith, T. C., Picco, A. M., and Knapp, R.: Ranaviruses infect mountain yellow-legged frogs (Rana muscosa and Rana sierrae) threatened by Batrachochytrium dendrobatidis, Herpetol. Conserv. Bio., 12, 149–159, 2017.
Soto-Azat, C., Peñafiel-Ricaurte, A., Price, S. J., Sallaberry-Pincheira, N., García, M. P., Alvarado-Rybak, M., and Cunningham, A. A.: Xenopus laevis and Emerging Amphibian Pathogens in Chile, EcoHealth, 13, 775–783, https://doi.org/10.1007/s10393-016-1186-9, 2016.
Stallknecht, D. E.: Impediments to wildlife disease surveillance, research, and diagnostics, in: Wildlife and emerging zoonotic diseases: the biology, circumstances and consequences of cross-species transmission, edited by: Childs, J. E., Mackenzie, J. S., and Richt, J. A., Springer, Berlin, Heidelberg, 315, 445–461, https://doi.org/10.1007/978-3-540-70962-6_17, 2007.
Standish, I., Leis, E., Schmitz, N., Credico, J., Erickson, S., Bailey, J., Kerby, J., Phillips, K., and Lewis, T.: Optimizing, validating, and field testing a multiplex qPCR for the detection of amphibian pathogens, Dis. Aquat. Organ., 129, 1–13, https://doi.org/10.3354/dao03230, 2018.
Stöhr, A. C., Hoffmann, A., Papp, T., Robert, N., Pruvost, N. B. M., Reyer, H.-U., and Marschang, R. E.: Long-term study of an infection with ranaviruses in a group of edible frogs (Pelophylax kl. esculentus) and partial characterization of two viruses based on four genomic regions, Vet. J., 197, 238–244, https://doi.org/10.1016/j.tvjl.2013.02.014, 2013.
Strachinis, I., Marschang, R., Lymberakis, P., Karagianni, K., and Azmanis, P.: Infectious disease threats to amphibians in Greece: new localities positive for Batrachochytrium dendrobatidis, Dis. Aquat. Organ., 152, 127–138, https://doi.org/10.3354/dao03712, 2022.
Talbott, K., Wolf, T., Sebastian, P., Abraham, M., Bueno, I., McLaughlin, M., Harris, T., Thompson, R., Pessier, A., and Travis, D.: Factors influencing detection and co-detection of Ranavirus and Batrachochytrium dendrobatidis in Midwestern North American anuran populations, Dis. Aquat. Organ., 128, 93–103, https://doi.org/10.3354/dao03217, 2018.
Thumsová, B., Price, S. J., González-Cascón, V., Vörös, J., Martínez-Silvestre, A., Rosa, G. M., Machordom, A., and Bosch, J.: Climate warming triggers the emergence of native viruses in Iberian amphibians, IScience, 25, 105541, https://doi.org/10.1016/j.isci.2022.105541, 2022.
Titus, V. R. and Green, T. M.: Presence of Ranavirus in Green frogs and Eastern Tiger salamanders on Long Island, New York, Herpetological Review, 44, 266–267, 2013.
Tornabene, B. J., Blaustein, A. R., Briggs, C. J., Calhoun, D. M., Johnson, P. T. J., McDevitt-Galles, T., Rohr, J. R., and Hoverman, J. T.: The influence of landscape and environmental factors on ranavirus epidemiology in a California amphibian assemblage, Freshwater Biol., 63, 639–651, https://doi.org/10.1111/fwb.13100, 2018.
Tornabene, B. J., Crespi, E., Traversari, B., Stemp, K., Breuner, C., Goldberg, C., and Hossack, B.: Low occurrence of ranavirus in the Prairie Pothole Region of Montana and North Dakota (USA) contrasts with prior surveys, Dis. Aquat. Organ., 147, 149–154, https://doi.org/10.3354/dao03640, 2021.
Une, Y., Kudo, T., Tamukai, K. I., and Murakami, M.: Epidemic ranaviral disease in imported captive frogs (Dendrobates and Phyllobates spp.), Japan, 2012: a first report, JMM Case Reports, 1, e001198, https://doi.org/10.1099/jmmcr.0.001198, 2014.
Urgiles, V. L., Ramírez, E. R., Villalta, C. I., Siddons, D. C., and Savage, A. E.: Three Pathogens Impact Terrestrial Frogs from a High-Elevation Tropical Hotspot, EcoHealth, 18, 451–464, https://doi.org/10.1007/s10393-021-01570-8, 2021.
von Essen, M., Leung, W. T. M., Bosch, J., Pooley, S., Ayres, C., and Price, S. J.: High pathogen prevalence in an amphibian and reptile assemblage at a site with risk factors for dispersal in Galicia, Spain, PLoS ONE, 15, e0236803, https://doi.org/10.1371/journal.pone.0236803, 2020.
Vörös, J., Herczeg, D., Papp, T., Monsalve-Carcaño, C., and Bosch, J.: First detection of Ranavirus infection in amphibians in Hungary, Herpetology Notes, 13, 213–217, 2020.
Wagner, M. B.: Medindo a ocorrência da doença: prevalência ou incidência?, J. Pediatr., 74, 157–162, 1998.
Warne, R. W., LaBumbard, B., LaGrange, S., Vredenburg, V. T., and Catenazzi, A.: Co-Infection by Chytrid Fungus and Ranaviruses in Wild and Harvested Frogs in the Tropical Andes, PLoS ONE, 11, e0145864, https://doi.org/10.1371/journal.pone.0145864, 2016.
Watters, J. L., Davis, D. R., Yuri, T., and Siler, C. D.: Concurrent Infection of Batrachochytrium dendrobatidis and Ranavirus among Native Amphibians from Northeastern Oklahoma, USA, J. Aquat. Anim. Health, 30, 291–301, https://doi.org/10.1002/aah.10041, 2018.
Wheelwright, N. T., Gray, M. J., Hill, R. D., and Miller, D. L.: Sudden mass die-off of a large population of wood frog (Lithobates sylvaticus) tadpoles in Maine, USA, likely due to Ranavirus, Herpetological Review, 45, 240–242, 2014.
Whitfield, S., Geerdes, E., Chacon, I., Ballestero Rodriguez, E., Jimenez, R., Donnelly, M., and Kerby, J.: Infection and co-infection by the amphibian chytrid fungus and ranavirus in wild Costa Rican frogs, Dis. Aquat. Organ., 104, 173–178, https://doi.org/10.3354/dao02598, 2013.
Whitfield, S., Alvarado-Barboza, G., Abarca, J., Zumbado-Ulate, H., Jimenez, R., and Kerby, J.: Ranavirus is widespread in Costa Rica and co-occurs with threatened amphibians, Dis. Aquat. Organ., 144, 89–98, https://doi.org/10.3354/dao03576, 2021.
Wirth, W., Lesbarrères, D., and Ariel, E.: Ten years of ranavirus research (2010–2019): an analysis of global research trends, FACETS, 6, 44–57, https://doi.org/10.1139/facets-2020-0030, 2021.
World Organisation for Animal Health: Infection with Ranavirus, in: Aquatic Animal Health Code, 8th edn., World Organisation for Animal Health, 69–88, 2021.
Wynne, F. J.: Detection of ranavirus in endemic and threatened amphibian populations of the Australian Wet Tropics Region, Pacific Conservation Biology, 26, 93, https://doi.org/10.1071/PC19009, 2020.
Zhang, M., Hu, Y. H., Xiao, Z. Z., Sun, Y., and Sun, L.: Construction and analysis of experimental DNA vaccines against megalocytivirus, Fish Shellfish Immun., 33, 1192–1198, 2012.