the Creative Commons Attribution 4.0 License.
the Creative Commons Attribution 4.0 License.
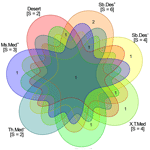
Pollen morphological variability correlates with a large-scale gradient of aridity
Hindel Fatmi
Souhaïl Mâalem
Bouchra Harsa
Ahmed Dekak
The study of the morphology of living organisms is essential to understand their evolution and diversity. This study aims to determine the importance of climatic gradients in the diversity of pollen morphotypes using Atriplex halimus L. (Amaranthaceae) as a model species. Atriplex halimus is a perennial shrubby plant, polymorphic and very resistant to severe environmental conditions. In seven bioclimatic zones, ranging from mesic conditions in the north to hot–hyperarid in the south, pollen samples were collected from 49 sites, with seven accessions per bioclimate. Under a light microscope, pollen grains were selected and analyzed from three anthers of different flowers. Besides the usual pollen grain types, some previously unknown morphotypes, such as sulcate, triangular, and ovoid, were observed and described at the different climatic zones. A total of 10 pollen grain shapes were quantified and discussed following their specific occurrences within different climatic zones. Occurrence frequencies of different pollen shapes ranged between 0 % and 85.7 %, where the pantoporate spheroidal was the most widespread in all climatic zones, especially in the desert climate (85.7 %). Five pollen types occurred exclusively once per climate zone. The pantoporate prolate spheroidal in mesomediterranean climate with a long dry season (28.6 %), sulcate in the xerothermomediterranean climate (14.3%), pantoporate subtriangular in the subdesert climate with a short dry season (14.3 %), and pantoporate subprolate and boat-shaped in a subdesert climate with a long dry season with 14.3 % for each. Our findings help to understand the evolutionary effects of climate gradients on pollen morphology and variability in arid and desert areas and point towards a high degree of specialization in order to maximize trade-offs between pollination efficiency and protection of pollen grains from dehydration.
- Article
(5192 KB) -
Supplement
(226 KB) - BibTeX
- EndNote
Pollen grain studies need to cover all phenotypical aspects (e.g., apertures, shapes, pores, dimensions, colors), polarity traits, and also cytoplasmic characteristics in order to efficiently resolve current palynological problems (species and varietal identification in the fields of botany, paleobotany, and forensic palynology) and thus advance our understanding of the biology of pollination (Muller, 1979; Prieu, 2015). Much scientific research has integrated pollen morphological diversity with the taxonomy of angiosperms (Mallick, 2019; Prieu et al., 2019). Pollen is involved in reproduction and is therefore subject to strong selection pressures, which along with the environmental constraints influence its morphology in both the short and long term (Young and Stanton, 1990a; Prieu, 2015). Some environmental and/or biotic stresses can affect the availability of resources to plants and thus reduce pollen production as a result of changes in the number of flowers and pollen production per flower. These stresses can be related to soil fertility (Lau et al., 1995; Havens et al., 1995), previous production of fruits and seeds (Delph, 1990; Young and Stanton, 1990a; Stephenson et al., 1994), mycorrhizal infection and effectiveness (Lau et al., 1995), and herbivory intensity (Quesada et al., 1995).
The current study focused on morphological diversity of pollen grains of the Mediterranean saltbush Atriplex halimus L. (Amaranthaceae) in steppe rangelands of Algeria, where the species stretches over about 10 000 km2 in the form of halophytic steppe vegetation (Macheroum and Kadik, 2015). In general, North African steppes in semiarid and arid regions are in a serious state of degradation due to the continuous deterioration of vegetation cover undergoing severe environmental forcings viz. drought and soil salinization, combined with serious human pressures, mainly overgrazing and cultivation of nonagricultural lands (Neffar et al., 2013, 2018; Kouba et al., 2020).
Atriplex halimus is an interesting species, because it divulges many characteristics of the reproductive system at the scale of the plant (Talamali et al., 2006) and is characterized by a significant diversity, on morphological and genetic scales (Ortiz-Dorda et al., 2005; Benzarti et al., 2013). The species adapts well to difficult environmental conditions with a high tolerance to salinity and drought (Neffar et al., 2016; Chenchouni, 2017). It is native in Europe, North Africa, and the Middle East, where it is used as an important plant for reclamation of degraded rangelands in arid and semiarid regions due to its high foraging value for livestock. The species has several medicinal virtues, for instance leaves can be used to treat heart diseases, diabetes, and rheumatism; it can also be used for phytoremediation and phyto-desalination of road runoff (Walker et al., 2014; Suaire et al., 2016).
According to the angiosperm phylogeny group (Müller and Borsch, 2005; Angiosperm Phylogeny Group, 2009), the pollen of Amaranthaceae species, especially A. halimus, is little studied. The available data, so far, included some inputs about number of pores, pollen grain diameter, and spinule and puncta densities (Hao et al., 1989; Flores Olvera, 1992). Mulder (1999) proposed a key to classify the Mediterranean types of pollen shapes in Amaranthaceae (formerly known as Chenopodiaceae) inhabiting drylands. Several studies suggested that phenotypic changes in plant organs can be fast in nature (Reznick and Ghalambor, 2001; Uyeda et al., 2011). We assume that the unusual morphological shapes are a priori due to plant adaptation to certain factors such as temperature, intra- and/or interspecific competition, phenotypic plasticity, and mutations (Delph, 1990). The latter may be at the origin of the appearance of certain types of pollen (Young and Stanton, 1990b).
The current survey aimed at studying pollen morphological diversity of A. halimus in arid and semiarid steppe rangelands of NE Algeria. It analyzed new pollen types encountered at the different bioclimatic zones of this region. The analysis included qualitative and quantitative comparisons of similarity and dissimilarity of pollen types between the climate regions surveyed.
2.1 Study area
This study was carried out in halophytic steppe rangelands, mostly dominated by A. halimus, growing wild in the region of Tébessa (34∘15′ to 35∘45′ N, 7∘30′ to 8∘30′ E) located in northeastern Algeria (Fig. 1). With an area of about 14 000 km2, this region rises about 960 m above sea level and is naturally connected with the immense steppe expanse of the country. It belongs to the semiarid bioclimatic zone of North Africa, located between the two Atlas mountain ranges, namely the Tellian mountains in the north and Saharan mountains in the south. A large climatic gradient exists from north to south over Tébessa region (Fig. 1), with more or less mesic conditions in the north and hot-hyperarid in the south (Mekahlia et al., 2013).
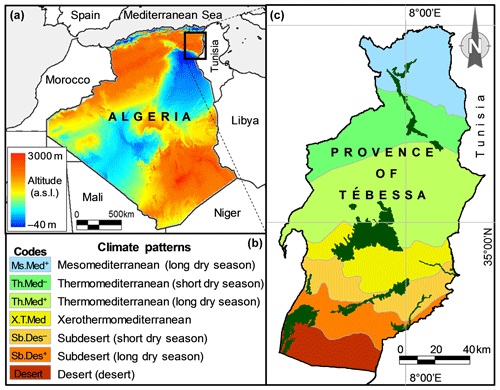
Figure 1Elevation map of Algeria (a) and map of bioclimate zones of Tébessa (c) with its legend (b), displaying locations in the study area and distribution of steppe rangelands of Atriplex halimus (forest-green color in right map) in the region of Tébessa, northeastern Algeria. The symbols “+” and “–” associated with climate codes in the map legend refer to short and long dry seasons of climates, respectively.
Based on long-term meteorological data (1972–2015) of Tébessa weather station, the average annual temperature was 15.5 ∘C and the average annual rainfall is about 371.2 mm. July was the hottest month with an average temperature of 27.3 ∘C, while the lowest temperature (6.4 ∘C) was recorded during January. September was the rainiest month (average monthly rainfall = 43.1 mm), whereas the driest month was July with an average of 14.37 mm (Fig. 2; Supplement, Table S1). The region of Tébessa is characterized by a semiarid rangeland vegetation type, principally dominated by Stipa tenacissima, Artemisia herba-alba, and Atriplex halimus. Lands are used mainly for the cultivation of rainfed cereal crops (durum, barley, and bread wheats) and livestock grazing (Djellab et al., 2019). Following Köppen's climatic classification, the arid climate of the region characterized either a cold steppe “BSk” or hot desert “BWh”, where precipitation deficit exceeded 800 mm yr−1 and limited the climatic net primary production (Table A1 in Appendix A).
2.2 Pollen sampling
Sampling was carried out in seven bioclimatic zones that characterize the region of Tébessa, ranging from mesomediterranean in the north to desert in the south (Fig. 1). Within each of the seven climatic zones studied, seven accessions of A. halimus were randomly sampled to study pollen morphology. A set of 20 pollen grains from each accession was selected from three anthers of different flowers that were collected from leafy and inflorescent twigs of native and wild-grown A. halimus plants. A total of 49 sites were sampled and analyzed (Table A2).
2.3 Pollen visualization
Anthers from each sample and of each accession were placed into drops of H2SO4 (95 %) on microscopic glass slides. The slides were heated over a candle flame for 10 s to mollify the anthers, release the pollen grains from anthers, and dissolve extra organic material on pollen grain surfaces (Grímsson et al., 2018). Randomly selected, 20 pollen grains from each anther were then transferred onto new slides for identification under a light microscope with 400× magnification. By referring to illustrated pollen terminology (Halbritter et al., 2018) and a glossary of pollen and spore terminology (Punt et al., 2007), types and shapes of pollen grains were sorted and characterized. We also used morphological pollen determination keys following the example of Erdtman (1952), who categorized eight shape classes based on the ratio of polar axis (PA) to equatorial diameter (ED). Based on the equatorial view, the ratio between the PA and ED, multiplying by 100 gives an accurate indication of pollen shape; accordingly, pollen types have been classified as shown in Table 1.
Table 1Pollen shape classes and suggested relationships between polar axis (PA) and equatorial diameter (ED) (Erdtman, 1952).
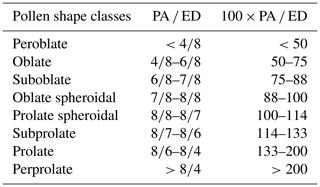
For some pollen grain types, such as “pantoporate”, it is not possible to distinguish where the proximal and distal poles are, and, thus, the PA and ED, the ratio of larger dimension to smaller dimension was employed instead of PA ∕ ED. Observations revealed the existence of pantoporate spheroidal globular types (usual pollen grains in Amaranthaceae) as well as exceptional shapes found at certain study sites such as triangular, boat-shaped, and sulcate (Fig. 3).
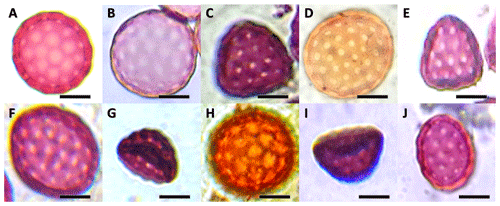
Figure 3Light micrographs showing equatorial views of pollen grains of the Atriplex halimus collected from arid and semiarid steppe rangelands of the region of Tébessa, northeastern Algeria. Pollen shapes identified: (A) pantoporate spheroidal, (B) pantoporate prolate spheroidal, (C) pantoporate triangular pyramid, (D) pantoporate oblate spheroidal, (E) pantoporate subtriangular, (F) pantoporate suboblate, (G) sulcate, (H) pantoaperturate spheroidal, (I) boat-shaped, and (J) pantoporate subprolate. Scale bar = 10 µm.
2.4 Data analysis
The number of pollen type occurrences per climatic zone was computed and displayed as a heatmap with two-way clustering analysis. Based on a contingency table summarizing the number of pollen types present at each climatic zone, a correspondence analysis (CA) was performed in order to distinguish the pollen types that characterize each zone and those that are common among them. The variation in occurrence odds of each pollen grain shape among the bioclimatic zones was tested using a generalized linear model (GLM) with a binomial distribution error and logit link. In addition, the effects of latitude, longitude, and altitude on the occurrence of each pollen grain shape were tested using a generalized linear mixed-effect model (GLMM), where geo-coordinates (latitude, longitude, and altitude) were the fixed effects and the bioclimatic zones were considered the random effect. Similarity of pollen morphotypes between climatic zones was analyzed qualitatively and quantitatively using the Jaccard coefficient and Bray–Curtis distance, respectively. Shared and zone-specific pollen shapes among the climatic zones were displayed using a seven-set Venn diagram. Statistical analyses were carried out using the R packages d3heatmap, nlme, and venn.
3.1 Spatial occurrences and distribution of pollen grains
Pollen occurrence frequency per climatic zone varied between 0 % and 85.7 % (Fig. 4). The most common pollen type in A. halimus among the climatic zones studied was the pantoporate spheroidal type that totaled 85.7 % of pollen occurrences at the desert climate. The lowest value of occurrence frequency of this type was recorded at Sb.Des− and Ms.Med+ with 28.6 %, followed by Th.Med− and Sb.Des+ zones with 42.9 %, and then Th.Med+ and X.T.Med zones with 57.1 %. As for the pantoaperturate spheroidal type, which was present in all studied climatic zones except Th.Med+ and desert, its occurrence was the highest at Th.Med− where it totaled 57.1 %. Occurrence frequencies of this pollen type were similar at Ms.Med+ and Sb.Des− (42.9 %) and at X.T.Med and Sb.Des+ (14.3 %). At Sb.Des+ pollen grains with pantoaperturate spheroidal, pantoporate oblate spheroidal, pantoporate subprolate, boat-shaped, and pantoporate suboblate types have the same occurrence frequency (14.3 %). It is worth mentioning that five pollen types occurred exclusively in one climate zone, namely the pantoporate prolate spheroidal type in Ms.Med+ (28.6 %), the sulcate type in X.T.Med (14.3 %) and pantoporate subtriangular type in Sb.Des− (14.3 %), and the pantoporate subprolate and boat-shaped types in Sb.Des+ with 14.3 % for each (Fig. 4).
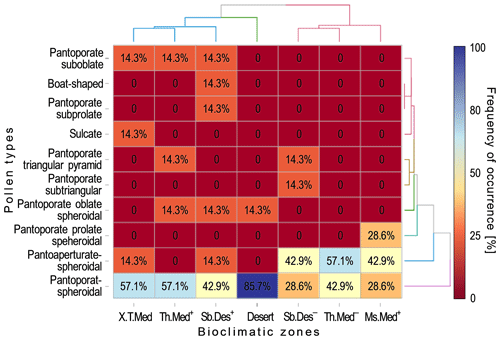
Figure 4Heatmap with two-way clustering analysis of pollen grain types of Atriplex halimus collected from seven bioclimatic zones in steppe rangelands of northeastern Algeria. Clustering was performed using absolute occurrences of pollen shapes, whereas figures associated with color intensity and displayed in the plot are relative frequency in percent. (See Fig. 1 for codes of the bioclimatic zones studied.)
3.2 Spatial variation in pollen grain occurrences
Results of GLMs testing the variation in pollen shape occurrences (presence or absence of data) among the bioclimatic zones showed that there was no significant difference in occurrence odds of all pollen aspects except for the pantoaperturate spheroidal type whose occurrences varied significantly between climatic regions (χ2=14.39, p=0.026) (Table 2). Furthermore, the GLMM testing effects of latitude, longitude, and altitude on the spatial occurrence of each pollen grain shape revealed that latitude had a negative effect on occurrence odds of all pollen aspects except for the pantoaperturate spheroidal type that was positive (GLMM: t=1.581, p=0.122); however all these effects were not statistically significant except for the pantoporate triangular pyramid type (, p=0.021) (Table 3). Spatial occurrence of the sulcate type was deemed negatively influenced by longitude (, p=0.022) and positively by altitude (t=2.047, p=0.047). Thus, the linear model predicting occurrence odds of the previous two pollen types following geo-coordinates in northeastern Algeria is expressed as follows:
with latitude and longitude expressed in decimal units and altitude in meters.
Table 2Generalized linear models (binomial distribution + logit link) testing the variation in each pollen grain type of Atriplex halimus among seven bioclimatic zones in steppes of northeastern Algeria (Df: degrees of freedom; χ2: chi-square value of likelihood ratio (LR) test).
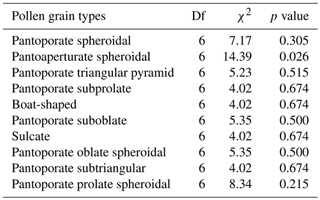
For the rest of pollen types, GLMMs indicated that effects of latitude, longitude, and altitude on the variation in spatial occurrences were not significant (Table 3).
3.3 Characteristic pollen shapes of bioclimatic zones
The correspondence analysis (CA) determined both characteristic and common pollen types of different climatic zones surveyed in NE Algeria (Fig. 5). The two axes of CA captured 57.48 % of the total variance. The pantoporate prolate spheroidal type was deemed to characterize the mesomediterranean climate with a long dry season and the pantoaperturate spheroidal type characterized the thermomediterranean climate with a short dry season. Pantoporate triangular pyramid and pantoporate subtriangular were the typical pollen types in the subdesert climate with a short dry season. The subdesert climate with a long dry season was characterized by pantoporate subprolate and boat-shaped types. Pantoporate oblate spheroidal, pantoporate suboblate, pantoporate spheroidal, and sulcate constituted a cluster that characterized mainly thermomediterranean with a long dry season, xerothermomediterranean, and desert climates.
3.4 Diversity and distribution of pollen grains
Following the Venn diagram, the pantaporate spheroidal type was the common pollen morphotype of A. halimus that was observed in all climatic zones (Fig. 6). Pantoaperturate spheroidal was common between five climatic zones, pantoporate suboblate and pantoporate oblate spheroidal were shared between three zones, and pantoporate triangular pyramid occurred exclusively in two zones. Three pollen types were exclusively specific to one climate zone: pantoporate prolate spheroidal type in Ms.Med+, sulcate in X.T.Med, and pantoporate subtriangular in Sb.Des−. The Sb.Des+ zone had two exclusive pollen grain types (pantoporate subprolate and pantoporate triangular pyramid).
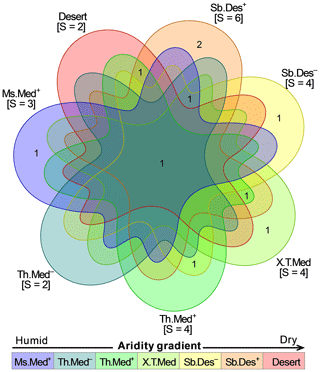
Figure 6Seven-set Venn diagram displaying the occurrence of different pollen types of Atriplex halimus among climatic zones in steppe rangelands of northeastern Algeria. (See Fig. 1 for codes of the bioclimatic zones studied.) Values between square brackets are the total number of pollen types “S” per climate.
3.5 Qualitative and quantitative similarity analysis
Based on Bray–Curtis distances (quantitative similarity), 11 out 21 pairwise comparisons revealed high similarity (>50 %) (Fig. 7). The highest similarity scores (71 %) were observed for the pairs Ms.Med+ and Th.Med−, Ms.Med+ and Sb.Des−, Th.Med− and Sb.Des−, Th.Med+ and X.T.Med, and Th.Med+ and desert. The lowest quantitative similarity (29 %) of pollen shapes climates zones was recorded between for the pairs Ms.Med+ and Th.Med+, Ms.Med+ and desert, and Sb.Des− and desert. The qualitative similarity analysis indicated low similarity between climatic zones where only 23.8 % of computed similarities showed values of Jaccard index greater than 50 %. The highest similarity scores (Jaccard = 66.7 %) were recorded between relatively mesic climates of the region, i.e., Ms.Med+ and Th.Med−, while the lowest similarity (16.7 %) was obtained between Ms.Med+ and Th.Med+.
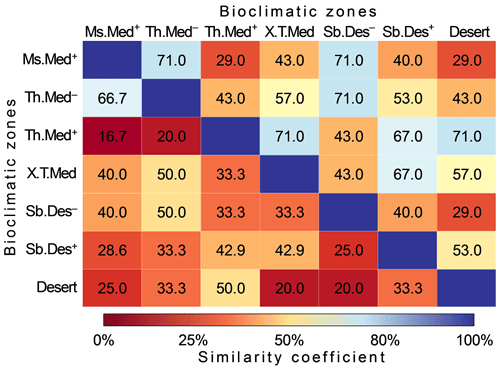
Figure 7Matrix of similarity of pollen grain types between seven climatic zones in Algeria. Values of similarity are displayed in color intensity proportionally to Bray and Curtis distance (above diagonal) and Jaccard' similarity coefficient (below diagonal). (See Fig. 1 for codes of the bioclimatic zones studied.)
This essay fundamentally treated the questions related to morphological diversity of pollen grains in different bioclimatic zones of Algeria (North Africa). The study investigated the reasons that led to the appearance of new pollen types in A. halimus collected from arid and semiarid steppe rangelands of Algeria. It is worth mentioning that studying pollen diversity contributes greatly to the enrichment of pollen libraries and thus helps to understand the mechanisms of taxonomic and functional speciation.
4.1 Patterns of pollen grains observed
The most frequent pollen type in A. halimus is pantoporate spheroidal which was encountered at all climatic zones studied. However, other pollen aspects and types of unprecedented sizes and shapes, such as triangular, boat-shaped, and sulcate with two tight poles, as well as different colors like orange, purple, pink, and yellow, have been recorded in all climate zones. This variation in pollen colors can be explained by chemical composition. Indeed, chemical studies of pollen pigments have shown that there are two main classes of components that determine pollen color: flavonoids and carotenoids, and there can be intraspecific variations in terms of pollen colors (Kearns and Inouye, 1993). Our findings highlighted the existence of intraplant polymorphism, since the exceptional pollen types were observed side by side with the common interplant and intersite shapes. The observed pollen grains of A. halimus in northeastern Algeria were of different colors and sizes (small, large, and intermediate), which corroborates with the results of Talamali et al. (2007), who studied two A. halimus populations in Tunisia, one from the region of Tadjerouine, located near our study area, where in addition to the three pollen sizes described above, orange- and yellow-colored pollen grains have been reported. The pantoaperturate spheroidal type, very close to the pantoporate spheroidal type, was also the most commonly observed with the latter.
4.2 Factors influencing the shape of pollen grains
Regarding the intraspecific pollen size variation, several studies have addressed the question with hypotheses in order to explain these evolutionary changes (Ejsmond et al., 2011). Beaulieu et al. (2008) proposed that polyploidy and/or genome sizes are predictors of larger sizes of pollen grains. According to Amer and Amany (2014), there is a solid relationship between polyploidy and pollen morphological variation in A. halimus, where the most touched morphological characters are exine features, pore membrane, number of pores, pore shape, spinule shape, and pore margin. However, Knight et al. (2010) revealed that there was no relationship between pollen size and genome size through controlling the phylogenetic history of 464 plant species.
Furthermore, abiotic stresses affect pollen development at almost all ecozones worldwide (Firon et al., 2012; Bokszczanin and Fragkostefanakis, 2013; Müller and Rieu, 2016; García et al., 2017). In fact, pollen grain size may vary depending on a bunch of environmental and biotic variables controlling the growing conditions (Muller, 1979; Young and Stanton, 1990a; Stephenson et al., 1994; Torres, 2000). Among these conditions, the air temperature and moisture are the most crucial factors (Ejsmond et al., 2011; Firon et al., 2012). Indeed, Ejsmond et al. (2011) stressed that the number of pollen produced as well as the optimization of its size are closely related to the ambient temperature, but the latter does not significantly affect its shape. Concerning the effects of moisture on pollen, pollen grain walls (intine and exine) can cope with changes in volume due to up-take or loss of water; they lose water, decreasing in volume, when kept in a dry environment, but gain water, increasing in volume, when kept under humid conditions (Pacini, 1990; Pacini and Franchi, 2020). On the other hand, the plasma membrane plays a very important role in regulating the inflow and outflow of water. Its structure changes according to the water content of the grain (Platt-Aloia et al., 1986; Tiwari et al., 1990). Different responses of the plasma membrane to relative moisture have been detected in plants of the same group but living in different habitats (Chaudhury and Shivanna, 1987).
Before the opening of the anthers, pollen grains endure a significant dehydration and generally lose about 15 %–35 % of cytoplasmic water. In addition, under certain conditions, this loss of water leads to a reduction in pollen grain volume and the wall folds back along the apertures (Nepi et al., 2001). Pollen grains are rehydrated once they reach a compatible stigma and increase in volume, leading to harmomegathy, which means changes in pollen volume accommodation in response to water loss (Wodehouse, 1965). According to Volkova et al. (2013), these harmonic changes occur when the cytoplasm and pollen grain walls collaborate to maintain protoplast viability, despite changes in shape and volume. This process is facilitated by pollen wall grooves (colpi) that facilitate the change in pollen grain shape after water loss.
Variations in the composition, organization, and shape of pollen grains are mainly due to harmomegathic stress (Muller, 1979; Payne, 1981). This could explain the pantoporate oblate spheroidal types of pollen that were observed in climatic zones 3, 6, and 7. In light of these results, it can be argued that the climate aridity and drought that characterize climatic zones 5 and 6 could influence the new pollen morphotypes (i.e., sulcate, triangular, and boat-shaped) found in these zones. Katifori et al. (2010) stipulate that in dry environments, the pollen wall has the ability to fold back on itself in order to prevent further losses of water, which is in agreement with the work of Muller (1979). These results were affirmed by Volkova et al. (2013), who testify that the pollen grains have developed the ability to bend in order to avoid dehydration, which could explain the appearance of the sulcate and pantoporate subtriangular types in climatic zones 4 and 5 of the study area. Moreover, plants coexisting in the same habitat with limited resources and/or under extreme conditions compete for the resources necessary for their growth. This competition also concerns pollen, where it can affect pollen phenotypes of offspring (Snow and Mazer, 1988) and pollen performance (Schlichting, 1986).
Furthermore, the change of pollination mode can influence pollen shape and size (Torres, 2000). In the same sense, Edlund et al. (2004) suggest that pollen grain size is the result of biotic and abiotic preference in pollinators. This preference is linked to diverse traits of pollen of angiosperms, including the diversity of nutrients available for pollinators (Roulston et al., 2000), the diversity of flavors (Dobson and Bergström, 2000), and pigments (Lunau, 2000). Regarding pollen colors, both carotenoids and flavonoids may cause a yellow pollen color. According to Stanley and Linskens (1974), pollen grains contain representatives of the colorless and yellow flavonoids; while most flavones and flavonols combine to yield yellow-colored pollen, some pollen is red or blue. Anthocyanins are generally the source of these colors. The amount of flavonoid, its location, and the presence of other pigments all contribute to the visible color of pollen, and the appearance of its extract. Small quantities of carotenoids can drastically modify the apparent color of pollen flavonoids. Identical flavonoids may be present in two species but because of variation in concentration and presence of co-pigments, the pollens may appear different in color. These diverse traits can also explain the different pollen colors (orange, yellow, pink, and purple) observed in this study. Moreover, it is important to mention that some flowers of angiosperms produce sterile pollen to attract and reward pollinators. For example, dimorphic anthers of Lagerstroemia indica produce fertile blue pollen and nourishing sterile yellow pollen. Although the nourishing pollen can germinate, its tubes never reach the style (Pacini and Bellani, 1986; Pacini and Franchi, 2020).
Other factors may lead to pollen phenotypic changes. Among these factors, altitude plays an important role in pollen morphological diversity. According to Prieu (2015), pollen grains with four apertures are more frequent at high elevations, and pollen grains with five apertures dominate at low elevations. The highest elevation in the study area is 1090 m recorded in zone 4 where the sulcate and pantoporate subprolate types were observed. In some plants, elevation affects pollination efficiency, which decreases with the increase in altitude (Till-Bottraud et al., 1999). This can be explained by the fact that pollinators are rarer at high altitudes, but this incites production of pollen that survives for a long time and thus reduces the vagaries of pollination (Prieu, 2015).
4.3 Heteromorphism and polymorphism
Pollen heteromorphism, i.e., the production of several fertile pollen formed by a single plant, is seen in about one in three families of angiosperms (Till et al., 1989). This study showed that some individuals of A. halimus produced different types of pollen “heteromorphism”. The production of several types of pollen can be advantageous in the long term, because each type is adapted to particular environmental conditions. This explains the high level of heteromorphism in flowering plants (Mignot et al., 1994). In a study involving 200 species (Mignot, 1995), no purely polymorphic species in regards to pollen has been detected. In addition, pollen variability can be influenced by the efficiency of transport by different pollinating insect species that can lead to evolutionary stability of pollen heteromorphism (Ejsmond et al., 2011).
4.4 Genetic aspects, phenotypic plasticity, and genotype–environment interaction
It is important to consider the genetic features to understand the appearance of certain shapes of pollen. In natural populations, genetic variations in pollen performance may result from mutations, gene flows among populations, and environmental heterogeneity within populations (Schlichting et al., 1990; Charlesworth and Charlesworth, 1992). Our results suggest the existence of intraindividual genetic variations, which are probably related to somatic mutations caused by the indefinite plant growth (Hallé, 2015). On the other hand, it seems unclear whether mutations are the only causes that could explain such a diversity of pollen. This leads us to suggest that these mutations can be, with the factors mentioned above, at the origin of a certain new type observed in this study such as the pantoporate prolate spheroidal type in the mesomediterranean climate with a long dry season, pantoporate subprolate grains in the subdesert climate with a short dry season, and pantoporate suboblate grains in xerothermomediterranean, thermomediterranean, and subdesert climates with a long dry season.
In addition, certain nongenetic pollen variations may correspond to phenotypic plasticity, which can be defined as the change of a character in response to a variation in the environment for a given genotype (Talamali et al., 2006; Lefèvre et al., 2015). This can explain the occurrence of certain pollen shapes as a response to environmental stresses and hazards. The triangular pyramid type is among these types that were recorded in the thermomediterranean (long dry season) and subdesert (short dry season) climates. The same is true for the boat-shaped type which was recorded in the subdesert (long dry season) climate. Accordingly, the following question arises: why did the majority of the observed pollen keep its usual shape? This could be explained by homeostasis, where a genotype maintains a stable character when the environment varies. There are three possible situations for a character to change following genotype and/or a range of environmental conditions: genetically non-variable plasticity (effect of the environment), absence of plasticity (no effect of the environment), or genetically variable plasticity (genotype × environment interaction). In the case of variable plasticity, the term “ecovalence” is also used, which refers to the contribution of a genotype to the genotype × environment interaction (Lefèvre et al., 2015).
The current study provided – for the first time – an extensive and comprehensive analysis of diversity and variations in pollen grain shapes of Atriplex halimus growing wild across a large climatic gradient in the steppes of Algeria and North Africa. New and previously unknown pollen types, with unique morphological characteristics such as grain size and shape were observed and described. The statistical modeling approach accompanied by qualitative and quantitative similarity analysis assisted in understanding spatial variations in pollen grains encountered in different bioclimatic zones in Algeria. Pollen morphological diversity is mainly attributed to abiotic factors such as air temperature that influence both size and shape of pollen grain, giving it the ability to fold back on itself to decrease dehydration. Other factors such as animal pollinators and altitude also play a role in pollen morphological variability. Addressing ecological variables behind pollen variability may explain morphophysiological adaptations of individuals and populations to climate change and unfavorable conditions. Carrying out genomic studies appears to be necessary in order to better understand the diversity of pollen recorded in this work. This can also be applied to other plant species of economic and medicinal interest.
Table A1Climatic characteristics of the zones in which arid and semiarid steppe rangelands of Atriplex halimus were studied in the region of Tébessa, NE Algeria.
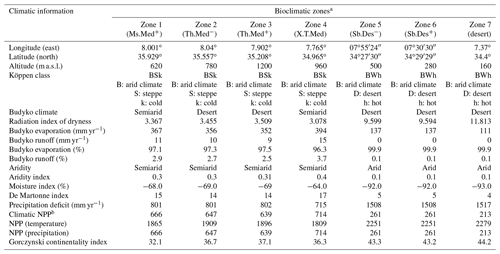
a See Fig. 1 for codes of the bioclimatic zones studied. b NPP: net primary production, in grams of dry matter per square meter per year, is precipitation limited.
The datasets used and analyzed during the current study are available from the corresponding author upon reasonable request.
The supplement related to this article is available online at: https://doi.org/10.5194/we-20-19-2020-supplement.
HF and SM conceived the study. HF conducted fieldwork, collected plant samples, carried out laboratory measurements, and compiled data. BH contributed to data collection. HC analyzed data and designed the paper. HF, HC, and AD wrote and revised the manuscript. All authors read and approved the final version of the manuscript.
The authors declare that they have no conflict of interest.
This paper was edited by Daniel Montesinos and reviewed by António Xavier Pereira Coutinho and two anonymous referees.
Amer, W. M. and Amany, S. A.: Infra-specific pollen diversity of Atriplex halimus L. in Egyptian flora, International Journal of Research Studies in Biosciences, 2, 36–48, 2014.
Angiosperm Phylogeny Group: An update of the Angiosperm Phylogeny Group classification for the orders and families of flowering plants: APG III, Bot. J. Linn. Soc., 161, 105–121, https://doi.org/10.1111/j.1095-8339.2009.00996.x, 2009.
Beaulieu, J. M., Leitch, I. J., Patel, S., Pendharkar, A., and Knight, C. A.: Genome size is a strong predictor of cell size and stomatal density in angiosperms, New Phytol., 179, 975–986. https://doi.org/10.1111/j.1469-8137.2008.02528.x, 2008.
Benzarti, M., Rejeb, K. B., Debez, A., and Abdelly, C.: Environmental and economical opportunities for the valorisation of the genus Atriplex: new insights, in: Crop Improvement, edited by: Hakeem, K., Ahmad, P., and Ozturk, M., Springer, Boston, MA, 441–457, https://doi.org/10.1007/978-1-4614-7028-1_16, 2013.
Bokszczanin, K. L. and Fragkostefanakis, S.: Solanaceae pollen thermotolerance initial training network C: Perspectives on deciphering mechanisms underlying plant heat stress response and thermotolerance, Front. Plant Sci., 4, 315, https://doi.org/10.3389/fpls.2013.00315, 2013.
Charlesworth, D. and Charlesworth, B.: The effects of selection in the gametophyte stage on mutational load, Evolution, 46, 703–720, https://doi.org/10.1111/j.1558-5646.1992.tb02077.x, 1992.
Chaudhury, R. and Shivanna, K. R.: Differential responses of Pennisetum and Secale pollen, Phytomorphology, 37, 181–185, 1987.
Chenchouni, H.: Edaphic factors controlling the distribution of inland halophytes in an ephemeral salt lake “Sabkha ecosystem” at North African semi-arid lands, Sci. Total Environ., 575, 660–671, https://doi.org/10.1016/j.scitotenv.2016.09.071, 2017.
Delph, L. F.: Sex-differential resource allocation patterns in the subdioecious shrub Hebe subalpina, Ecology, 71, 1342–1351, https://doi.org/10.2307/1938271, 1990.
Djellab, S., Mebarkia, N., Neffar, S., and Chenchouni, H.: Diversity and phenology of hoverflies (Diptera: Syrphidae) in pine forests (Pinus halepensis Miller) of Algeria, J. Asia-Pac. Entomol., 22, 766–777, https://doi.org/10.1016/j.aspen.2019.05.012, 2019.
Dobson, H. E. and Bergström, G.: The ecology and evolution of pollen odors, Plant Syst. Evol., 222, 63–87, https://doi.org/10.1007/bf00984096, 2000.
Edlund, A. F., Swanson, R., and Preuss, D.: Pollen and stigma structure and function: the role of diversity in pollination, Plant Cell, 16, S84–S97, https://doi.org/10.1105/tpc.015800, 2004.
Ejsmond, M. J., Wrońska-Pilarek, D., Ejsmond, A., Dragosz-Kluska, D., Karpińska-Kołaczek, M., Kołaczek, P., and Kozłowski, J.: Does climate affect pollen morphology? Optimal size and shape of pollen grains under various desiccation intensity, Ecosphere, 2, 1–15, https://doi.org/10.1890/ES11-00147.1, 2011.
Erdtman, G.: Pollen Morphology and Plant Taxonomy. Angiosperms, Almkvist & Wiksell, Stockholm, 1952.
Firon, N., Nepi, M., and Pacini, E.: Water status and associated processes mark critical stages in pollen development and functioning, Ann. Bot., 109, 1201–1214, https://doi.org/10.1093/aob/mcs070, 2012.
Flores Olvera, H.: Taxonomía del grupo Atriplex pentandra (Chenopodiaceae), Anales del Instituto de Biología Serie Botánica, 63, 155–194, 1992.
García, C. C., Nepi, M., and Pacini, E.: It is a matter of timing: asynchrony during pollen development and its consequences on pollen performance in angiosperms – a review, Protoplasma, 254, 57–73, https://doi.org/10.1007/s00709-016-0950-6, 2017.
Grímsson, F., Grimm, G. W., and Zetter, R.: Evolution of pollen morphology in Loranthaceae, Grana, 57, 16–116, https://doi.org/10.1080/00173134.2016.1261939, 2018.
Halbritter, H., Ulrich, S., Grímsson, F., Weber, M., Zetter, R., Hesse, M., Buchner, R., Svojtka, M., and Frosch-Radivo, A.: Illustrated pollen terminology, Springer, Cham, https://doi.org/10.1007/978-3-319-71365-6, 2018.
Hallé, F.: Eloge de la plante. Pour une nouvelle biologie, Le Seuil, Paris, 2015.
Hao, H. P., Zhang, J. T., and Yan, S.: Scanning electron microscope observation on the pollen grains of Chenopodiaceae, Acta Bot. Sin., 31, 650–652, 1989.
Havens, K., Preston, K. A., Richardson, C., and Delph, L. F.: Nutrients affect allocation to male and female function in Abutilon theophrasti (Malvaceae), Am. J. Bot., 82, 726–733, https://doi.org/10.1002/j.1537-2197.1995.tb15683.x, 1995.
Katifori, E., Alben, S., Cerda, E., Nelson, D. R., and Dumais, J.: Foldable structures and the natural design of pollen grains, P. Natl. Acad. Sci. USA, 107, 7635–7639, https://doi.org/10.1073/pnas.0911223107, 2010.
Kearns, C. A. and Inouye, D. W.: Techniques for pollination biologists, University press of Colorado, Boulder, CO, USA, 1993.
Knight, C. A., Clancy, R. B., Götzenberger, L., Dann, L., and Beaulieu, J. M.: On the relationship between pollen size and genome size, Journal of Botany, 2010, 612017, https://doi.org/10.1155/2010/612017, 2010.
Kouba, Y., Merdes, S., Saadali, B., and Chenchouni, H.: Responses of individual plant species, functional groups, α- and β-diversity to short-term grazing exclusion under severe drought episode in long-term grazed alfa-steppes, Preprints, 2020010038, https://doi.org/10.20944/preprints202001.0038.v1, 2020.
Lau, T. C., Lu, X., Koide, R. T., and Stephenson, A. G.: Effects of soil fertility and mycorrhizal infection on pollen production and pollen grain size of Cucurbita pepo (Cucurbitaceae), Plant Cell Environ., 18, 169–177, https://doi.org/10.1111/j.1365-3040.1995.tb00350.x, 1995.
Lefèvre, F., Fady, B., Jean, F., Davi, H., Pichot, C., and Oddou-Muratorio, S.: Les processus biologiques de réponse des arbres et forêts au changement climatique: adaptation et plasticité phénotypique, Innovations Agronomiques, 47, 63–79, 2015.
Lunau, K.: The ecology and evolution of visual pollen signals, Plant Syst. Evol., 222, 89–111, https://doi.org/10.1007/BF00984097, 2000.
Macheroum, A. and Kadik, L.: Étude de l'étude actuel de la végétation du Nord de la wilaya de Tébessa sur le plan phytoécologie et pastoral, Edilivre, Paris, France, 2015.
Mallick, P. K.: Morphological Study of Pollen Grains of Angiosperms, International Journal of Applied Sciences and Biotechnology, 7, 354–358, https://doi.org/10.3126/ijasbt.v7i3.25714, 2019.
Mekahlia, M. N., Beddiar, A., and Chenchouni, H.: Mycorrhizal dependency in the olive tree (Olea europaea) across a xeric climatic gradient, Advances in Environmental Biology, 7, 2166–2175, 2013.
Mignot, A.: Contraintes et sélection dans l'évolution: le cas du pollen, Doctoral thesis, Univ. Tours, Tours, France, 1995.
Mignot, A., Hoss, C., Dajoz, I., Leuret, C., Henry, J. P., Dreuillaux, J. M., Heberle-Bors, E., and Till-Bottraud, I.: Pollen aperture polymorphism in the angiosperms: importance, possible causes and consequences, Acta Bot. Gallica, 141, 109–122, https://doi.org/10.1080/12538078.1994.10515144, 1994.
Mulder C.: Biogeographic re-appraisal of the Chenopodiaceae of Mediterranean drylands: A quantitative outline of their general ecological significance in the Holocene, Palaeoecol. Afr., 26, 161–188, 1999.
Müller, F. and Rieu, I.: Acclimation to high temperature during pollen development, Plant Reprod., 29, 107–118, https://doi.org/10.1007/s00497-016-0282-x, 2016.
Muller, J.: Form and function in angiosperm pollen, Ann. Mo. Bot. Gard., 66, 593–632, https://doi.org/10.2307/2398913, 1979.
Müller, K. and Borsch, T.: Phylogenetics of Amaranthaceae based on matK/trnK sequence data: evidence from parsimony, likelihood, and Bayesian analyses, Ann. Mo. Bot. Gard., 92, 66–102, 2005.
Neffar, S., Chenchouni, H., Beddiar, A., and Redjel, N.: Rehabilitation of Degraded Rangeland in Drylands by Prickly Pear (Opuntia ficus-indica L.) Plantations: Effect on Soil and Spontaneous Vegetation, Ecologia Balkanica, 5, 63–83, 2013.
Neffar, S., Chenchouni, H., and Si Bachir, A.: Floristic composition and analysis of spontaneous vegetation of Sabkha Djendli in North-east Algeria, Plant Biosyst., 150, 396–403, https://doi.org/10.1080/11263504.2013.810181, 2016.
Neffar, S., Menasria, T., and Chenchouni, H.: Diversity and functional traits of spontaneous plant species in Algerian rangelands rehabilitated with prickly pear (Opuntia ficus-indica L.) plantations, Turk. J. Bot., 42, 448–461, https://doi.org/10.3906/bot-1801-39, 2018.
Nepi, M., Franchi, G. G., and Padni, E.: Pollen hydration status at dispersal: cytophysiological features and strategies, Protoplasma, 216, 171, https://doi.org/10.1007/bf02673869, 2001.
Ortiz-Dorda, J., Martínez-Mora, C., Correal, E., Simón, B., and Cenis, J. L.: Genetic structure of Atriplex halimus populations in the Mediterranean Basin, Ann. Bot., 95, 827–834, https://doi.org/10.1093/aob/mci086, 2005.
Pacini, E.: Harmomegathic characters of Pteridophyta spores and Spermatophyta pollen, in: Morphology, Development, and Systematic Relevance of Pollen and Spores, edited by: Hesse, M. and Ehrendorfer F., Plant Systematics and Evolution, Vol. 5, Springer, Vienna, 53–69, https://doi.org/10.1007/978-3-7091-9079-1_5, 1990.
Pacini, E. and Bellani L. M.: Lagerstroemia indica L. pollen: form and function, in: Pollen and Spores. Form and Function, edited by: Blackmore, S. and Ferguson, I. K., Linnean Society Symposium Series, No. 12, Academic Press, London, UK, 347–357, 1986.
Pacini, E. and Franchi, G. G.: Pollen biodiversity – why are pollen grains different despite having the same function? A review, Bot. J. Linn. Soc., boaa014, https://doi.org/10.1093/botlinnean/boaa014, 2020.
Payne, W. W.: Structure and function in angiosperm pollen wall evolution, Rev. Palaeobot. Palyno., 35, 39–59, https://doi.org/10.1016/0034-6667(81)90013-0, 1981.
Platt-Aloia, K. A., Lord, E. M., DeMason, D. A., and Thomson, W. W.: Freeze-fracture observations on membranes of dry and hydrated pollen from Collomia, Phoenix and Zea, Planta, 168, 291–298, https://doi.org/10.1007/bf00392352, 1986.
Prieu, C.: Evolution et développement des grains de pollen chez les Angiospermes, Doctoral dissertation, Paris-Saclay University, 2015.
Prieu, C., Toghranegar, Z., Gouyon, P. H., and Albert, B.: Microsporogenesis in angiosperms producing pantoporate pollen, Botany Letters, 166, 457–466, https://doi.org/10.1080/23818107.2019.1652849, 2019.
Punt, W., Hoen, P. P., Blackmore, S., Nilsson, S., and Le Thomas, A.: Glossary of pollen and spore terminology, Rev. Palaeobot. Palyno., 143, 1–81, https://doi.org/10.1016/j.revpalbo.2006.06.008, 2007.
Quesada, M., Bollman, K., and Stephenson, A. G.: Leaf damage decreases pollen production and hinders pollen performance in Cucurbita texana, Ecology, 76, 437–443, https://doi.org/10.2307/1941202, 1995.
Reznick, D. N. and Ghalambor, C. K.: The population ecology of contemporary adaptations: what empirical studies reveal about the conditions that promote adaptive evolution, in: Microevolution rate, pattern, process. Contemporary Issues in Genetics and Evolution, edited by: Hendry, A. P. and Kinnison, M. T., Vol. 8, Springer, Dordrecht, 183–198, https://doi.org/10.1007/978-94-010-0585-2_12, 2001.
Roulston, T. A. H., Cane, J. H., and Buchmann, S. L.: What governs protein content of pollen: pollinator preferences, pollen–pistil interactions, or phylogeny?, Ecol. Monogr., 70, 617–643, https://doi.org/10.1890/0012-9615(2000)070[0617:wgpcop]2.0.co;2, 2000.
Schlichting, C. D.: Environmental stress reduces pollen quality in Phlox: compounding the fitness deficit, in: Biotechnology and ecology of pollen, edited by: Mulcahy, D. L., Mulcahy, G. B., and Ottaviano, E., Springer, NY, 483–488, https://doi.org/10.1007/978-1-4613-8622-3_78, 1986.
Schlichting, C. D., Stephenson, A. G., Small, L. E., and Winsor, J. A.: Pollen loads and progeny vigor in Cucurbita pepo: the next generation, Evolution, 44, 1358–1372, https://doi.org/10.1111/j.1558-5646.1990.tb05238.x, 1990.
Snow, A. A. and Mazer, S. J.: Gametophytic selection in Raphanus raphanistrum: a test for heritable variation in pollen competitive ability, Evolution, 42, 1065–1075, https://doi.org/10.1111/j.1558-5646.1988.tb02524.x, 1988.
Stanley, R. G. and Linskens, H. F.: Pollen pigments, in: Pollen: biology biochemistry management. Springer, Berlin, Heidelberg, 223–246, https://doi.org/10.1007/978-3-642-65905-8_15, 1974.
Stephenson, A. G., Erickson, C. W., Lau, T. C., Quesada, M. R., and Winsor, J. A.: Effects of growing conditions on the male gametophyte, in: Pollen–pistil interactions and pollen tube growth, edited by: Stephenson, A. G. and Kao, T.-H., Vol. 12, Current Topics in Plant Physiology. An American Society of Plant Physiologists Series, Rockville, Maryland, USA, 220–229, 1994.
Suaire, R., Durickovic, I., Framont-Terrasse, L., Leblain, J. Y., De Rouck, A. C., and Simonnot, M. O.: Phytoextraction of Na+ and Cl− by Atriplex halimus L. and Atriplex hortensis L.: A promising solution for remediation of road runoff contaminated with deicing salts, Ecol. Eng., 94, 182–189, https://doi.org/10.1016/j.ecoleng.2016.05.055, 2016.
Talamali, A., Gorenflot, R., Kinet, J. M., and Dutuit, P.: Floral plasticity and flower evolution in Atriplex halimus L. (Amaranthaceae), Acta Bot. Gallica, 153, 243–248, https://doi.org/10.1080/12538078.2006.10515540, 2006.
Talamali, A., Gorenflot, R., and Dutuit, P.: Hétérostylie intra-individuelle chez Atriplex halimus L. (Amaranthaceae), C. R. Biol., 330, 871–879, https://doi.org/10.1016/j.crvi.2007.09.003, 2007.
Till, I., Valdeyron, G., and Gouyon, P. H.: Polymorphisme pollinique et polymorphisme génétique, Can. J. Botany, 67, 538–543, https://doi.org/10.1139/b89-075, 1989.
Till-Bottraud, I., Vincent, M., Dajoz, I., and Mignot, A.: Pollen aperture heteromorphism Variation in pollen-type proportions along altitudinal transects in Viola calcarata, C. R. Acad. Sci.-Vie, 322, 579–589, https://doi.org/10.1016/s0764-4469(00)88528-5, 1999.
Tiwari, S. C., Polito, V. S., and Webster, B. D.: In dry pear (Pyrus communis L.) pollen, membranes assume a tightly packed multilamellate aspect that disappears rapidly upon hydration, Protoplasma, 153, 157–168, https://doi.org/10.1007/bf01354000, 1990.
Torres, C.: Pollen size evolution: correlation between pollen volume and pistil length in Asteraceae, Sex. Plant Reprod., 12, 365–370, https://doi.org/10.1007/s004970000030, 2000.
Uyeda, J. C., Hansen, T. F., Arnold, S. J., and Pienaar, J.: The million-year wait for macroevolutionary bursts, P. Natl. Acad. Sci. USA, 108, 15908–15913, https://doi.org/10.1073/pnas.1014503108, 2011.
Volkova, O. A., Severova, E. E., and Polevova, S. V.: Structural basis of harmomegathy: evidence from Boraginaceae pollen, Plant Syst. Evol., 299, 1769–1779, https://doi.org/10.1007/s00606-013-0832-8, 2013.
Walker, D. J., Lutts, S., Sánchez-García, M., and Correal, E.: Atriplex halimus L.: Its biology and uses, J. Arid Environ., 100, 111–121, https://doi.org/10.1016/j.jaridenv.2013.09.004, 2014.
Wodehouse, R. P.: Pollen grains, 3rd Edn., Hafner Publishing Co., New York & London, 1965.
Young, H. J. and Stanton, M. L.: Influence of environmental quality on pollen competitive ability in wild radish, Science, 248, 1631–1633, https://doi.org/10.1126/science.248.4963.1631, 1990a.
Young, H. J. and Stanton, M. L.: Temporal patterns of gamete production within individuals of Raphanus sativus (Brassicaceae), Can. J. Botany, 68, 480–486, https://doi.org/10.1139/b90-064, 1990b.