the Creative Commons Attribution 4.0 License.
the Creative Commons Attribution 4.0 License.
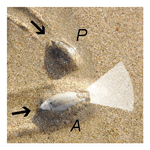
Short communication: Problems of classifying predator-induced prey immobility – an unexpected case of post-contact freezing
Teresita N. Ulate Gómez
Antonia E. Welch
Winfried S. Peters
Prey noticing predators may turn immobile to avert detection. Such “freezing” is generally thought to precede direct predator–prey contact and thus appears distinct from so-called “post-contact immobility”, or thanatosis. This distinction, however, may be inapplicable if predators lack long-distance senses or monitor their surroundings incompletely. The predatory sandy beach gastropod Agaronia propatula (Olividae) detects its prey, Pachyoliva semistriata of the same family, only when located within centimetres in front of the predator. Pachyoliva therefore reduces its risks by active flight when directly approached by an Agaronia, whereas it freezes to remain undetected when contacting Agaronia from the side. This unexpected “post-contact freezing” suggests that physical contact between prey and predator as such does not always help to distinguish freezing from other types of predator-induced prey immobility and highlights how our interpretations of predator–prey interactions depend critically on our understanding of the sensory capabilities involved.
- Article
(1828 KB) - Full-text XML
- BibTeX
- EndNote
Potential prey responds to predators by a variety of defensive behaviours including transient immobility (Edmunds, 1974; Caro, 2005; Cooper and Blumstein, 2018). Cases of predator-induced prey immobility fall into at least two categories that have not always been clearly distinguished (Sakai, 2021). Immobile states animals assume after becoming aware of a predator can help to remain cryptic and thus decrease the probability of being attacked (Eilam, 2005; Chelini et al., 2009; De Franceschi et al., 2016). This type of immobility is called “freezing”. Prey may fall into a different type of immobile state after it has been identified by the predator and physical contact has been made. This state, referred to as thanatosis, death-feigning, tonic immobility, catatonia, or animal hypnosis (Rogers and Simpson, 2014), has been documented in numerous vertebrates and invertebrates and probably reduces prey mortality through a variety of mechanisms that are only partly understood (Miyatake et al., 2004; Honma et al., 2006; Ruxton, 2006; Humphreys and Ruxton, 2018; Honma, 2021). To remedy the somewhat confusing terminology, it recently was suggested to subsume thanatosis, death-feigning, etc. under the term “post-contact immobility” (Sendova-Franks et al., 2020).
In the context of widely accepted concepts of the temporal progression of predator–prey interactions (Edmunds, 1974; Endler, 1986, 1991), freezing represents a primary and post-contact immobility a secondary defence response (Honma, 2021). Since in predator–prey interactions the physical distance between predator and prey usually decreases over time, and because freezing occurs earlier than post-contact immobility, it has been concluded – implicitly, in some cases – that freezing generally is performed before predator and prey come into contact, whereas physical contact induces post-contact immobility by definition (Misslin, 2003; Humphreys and Ruxton, 2018; Sendova-Franks et al., 2020; Honma, 2021; Carli and Farabollini, 2022). This notion, however, is based on tacit assumptions concerning the predator's sensory system. When predators lack long-distance sensory capabilities or monitor only limited parts of their immediate surroundings, physical contact between predator and prey as such may not be a valid criterion for distinguishing the maintenance of crypsis by freezing from other types of immobility.
The gastropod Pachyoliva semistriata (Olividae; formerly Olivella semistriata; Pastorino and Peters, 2023) is a common intertidal suspension feeder on sandy beaches of the Central American west coast (Troost et al., 2012). Its only known predator is an Agaronia species (tentatively identified as A. propatula) of the same family, whose prey spectrum Pachyoliva dominates (Robinson and Peters, 2018). As in other olivids (Marcus and Marcus, 1959; Kantor and Tursch, 2001), Agaronia's sensory capabilities appear restricted to the perception of mechanical and olfactory stimuli that originate from a narrow zone around its propodium (most anterior part of the foot; compare Fig. 1a; Cyrus et al., 2012). These limitations must be expected to affect the evolution of avoidance and escape strategies in Agaronia's prey species.
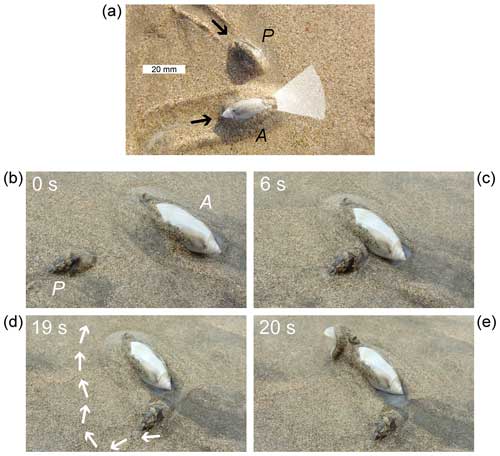
Figure 1Pachyoliva freezes upon contacting Agaronia laterally. (a) Agaronia (A) can localize the sources of olfactory and mechanical stimuli only in a narrow zone (white shade) before its propodium. When Pachyoliva (P) approaches it from the side, Agaronia is unlikely to become aware of the potential prey. (b–e) Frames extracted from Video 3 in the Supplement (time indicated in seconds), showing a misguided attack following lateral contact between Agaronia (A) and Pachyoliva (P). Pachyoliva approaches a moving Agaronia laterally (b) and freezes upon contact (c). Agaronia continues crawling, while surface water flows (arrows) around the frozen Pachyoliva towards the predator's sensitive anterior end (d). Eventually Agaronia performs a forward-directed attack strike (e).
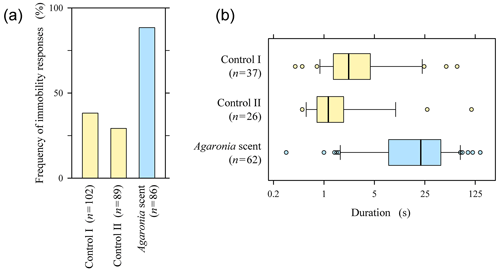
Figure 2Responses of crawling Pachyoliva to empty Turritella shells placed in their paths (Control I), empty Turritella shells with droplets of seawater added when Pachyoliva arrived (Control II), and empty Turritella shells to which droplets of Agaronia-scented seawater were added (Agaronia scent; n, number of biological replicates). (a) Frequencies of contact-induced immobility, defined as the lack of visible movement of the Pachyoliva shell. (b) Durations of the immobility responses reported in panel (a); not all cases could be analysed because incoming waves terminated some observations before Pachyoliva regained mobility. Boxes represent the second and third quartiles with the median marked by a bold line and the 10th and 90th percentiles shown by whiskers. Note the logarithmic time axis. Original data are available online in the Supplement; see Videos 4 (controls) and 5 (Agaronia scent) for representative results.
Pachyoliva exhibits various defence responses to Agaronia (for a video introducing commonly observed behaviour, see Peters, 2022). When approached by Agaronia directly, Pachyoliva switches to sculling, an accelerated mode of locomotion, and often outruns the attacking predator. Such active flight is triggered by mechanical stimuli (Veelenturf and Peters, 2020). Alternatively, Pachyoliva stops all movement when encountering Agaronia tracks on the sediment or is exposed to unidentified Agaronia-derived scent substances (Peters, 2023). This behaviour has the characteristics of freezing because, first, it is induced by the prey's perception of a nearby predator, and second, it can prevent the detection of the prey by the predator through mechanical cues. As shown in the following, Pachyoliva may also remain cryptic to its predator by freezing after direct physical contact has been made.
Field observations of natural behaviour made on the beach intertidal at Playa Grande, Costa Rica (10°20′ N, 085°51′ W) since 2011 were documented in writing and, if possible, filmed with waterproof digital cameras (e.g. Nikon Coolpix AW130).
To characterize responses of Pachyoliva semistriata to obstacles in its path, field experiments were conducted in July and August 2023 at Playa Grande in daylight between 2 h before to 1 h after low tide during the spring-tide phase. Empty shells of Turritella sp., available on the beach in large numbers, were positioned about 3 cm before crawling Pachyoliva (Control I). In a second set of tests, a few droplets of seawater were released with disposable plastic pipettes on top of the Turritella shell at the time Pachyoliva reached it (Control II), while in a third set, seawater carrying Agaronia-derived odours was used (Agaronia treatment). The Agaronia-scented medium was prepared by keeping 4–5 Agaronia (< 30 mm shell length) in 50 mL disposable centrifuge plastic tubes with ∼ 25 mL seawater for at least 10 min (compare Peters, 2023). All tests were filmed. The duration of any immobility responses was determined with 0.1 s accuracy by trimming the videos to the period in which no movement of the animal's shell was visible (QuickTime v7.7.9; https://www.apple.com/, last access: 12 March 2024). Statistical tests (Fisher's exact probability (FEP) test; Mann–Whitney test) were performed online on http://vassarstats.net/ (last access: 12 March 2024) (for details, see below).
No animals were killed, injured, or permanently collected in the course of this field study.
3.1 Field observations
In their beach habitat, crawling Pachyoliva sometimes collide with objects such as empty shells, pebbles, etc. In most cases, the snails temporarily slow down upon contact but immediately turn to move around the obstacle, start to burrow underneath it, or attempt to push the obstacle away. Occasionally, however, a snail's reaction is the cessation of all motion; the snail does not withdraw into its shell but rather maintains the body posture it had before the response commenced. Such immobility in response to inanimate objects usually lasts a few seconds. In contrast, when a Pachyoliva runs into an Agaronia laterally (Fig. 1a), it remains motionless until the predator has moved away (Video 1 in the Supplement), which may take minutes.
A thin water film often moves on the sediment where Pachyoliva turns immobile upon contacting a foraging Agaronia (Video 2 in the Supplement). If that water film flows in the direction in which the predator crawls, scents originating from the immobile Pachyoliva may enter the field that Agaronia monitors for mechanical and olfactory stimuli. In such cases, Agaronia may conduct an attack strike that obviously misses the target, as documented in Fig. 1b–e and Video 3 in the Supplement.
3.2 Field experiment
By definition, freezing is a response to becoming aware of a predator. To determine the extent to which contact-induced immobility in Pachyoliva is a specific response to the predator Agaronia, we placed empty Turritella shells collected on the beach with or without additional Agaronia-derived odours into the paths of crawling Pachyoliva. Pachyoliva running into Turritella shells turned immobile (i.e. movements of the shell ceased entirely) in roughly one-third of the cases (Fig. 2a, Control I; for examples, see Video 4a in the Supplement). This proportion did not increase when droplets of seawater were added to the Turritella shell at the time Pachyoliva arrived (Fig. 2a, Control II; Video 4b in the Supplement), demonstrating that the dropping as such did not induce defensive behaviour. In contrast, the proportion of immobility responses more than doubled when droplets of seawater in which Agaronia had been kept were added (Fig. 2a, Agaronia scent; Video 5 in the Supplement). The difference between the Agaronia-scent treatment and each of the two control experiments was highly significant statistically (, FEP test).
The median durations of the immobility responses in the two control experiments were below 2.5 s, with 84 % of the responses in the control tests combined showing durations of under 5 s (Fig. 2b). The median duration was 10 times higher when Agaronia-scented seawater had been added, with 77 % of the responses lasting for over 5 s (Fig. 2b). Differences between the Agaronia-scented treatment and each control treatment were highly significant statistically (, Mann–Whitney test).
The differentiation of various types of predator-induced immobility is notoriously problematic, which is at least partly due to terminological inconsistencies. For instance, Asakura et al. (2022, p. 3) recently distinguished “freezing” from “death feigning” in beetles solely by the posture that the prey assumed and found that “when attacked … the prey would either feign death or freeze”. When prey responds to a predator's attack, both actors obviously are aware of each other. Consequently, the behaviour termed “freezing” cannot have served to prevent detection in this case. To avoid misunderstandings, we emphasize that here we adhere to the functional definition of freezing as “immobility used to reduce the risk of predator detection or tracking” (Humphreys and Ruxton, 2018, p. 2), which appears to be accepted by a majority of researchers in the field.
Pachyoliva identifies its predator Agaronia olfactorily, as it freezes specifically in response to water-borne Agaronia scents (Peters, 2023). On the other hand, crawling Pachyoliva sometimes becomes immobile transiently upon contact with random objects. The frequency of this response as well as the duration of immobility increased significantly when Agaronia-derived odours were present (Fig. 2). This suggests that Pachyoliva may perform “tentative freezing” when encountering an obstacle but that a full freeze response is evoked only when additional olfactory cues indicate the presence of a predator.
The crawling of Agaronia on the intertidal plain reliably indicates predatory motivation. Crawling Agaronia will attack literally every moving object in front of their propodium, including human fingers, empty gastropod shells, or pencils gently vibrating on the sediment surface (Cyrus et al., 2012; see Peters, 2022, for video documentation). Therefore it is not surprising that Pachyoliva takes to active flight by sculling when noticing an approaching Agaronia (Veelenturf and Peters, 2020). In contrast, when an Agaronia's trajectory is not directed towards Pachyoliva (as in Fig. 1, Videos 1–3 in the Supplement), Pachyoliva will not flee but freeze. This behavioural dichotomy appears beneficial for Pachyoliva due to the limitations of the sensory powers of Agaronia, which monitors a few square centimetres in front of its propodium only (Cyrus et al., 2012). Thus, if Pachyoliva senses an approaching object, it might already be targeted by a charging Agaronia, and immediate flight will be the best option. However, flight by sculling is expensive energetically, and Pachyoliva can only sustain this mode of locomotion for a few seconds (Veelenturf and Peters, 2020). Moreover, because Agaronia is mechano-sensitive (Cyrus et al., 2012), it may become aware of a previously unnoticed Pachyoliva that suddenly initiates high-intensity movements. Therefore, turning motionless when sensing an object that is not approaching or not moving at all appears the most appropriate action for Pachyoliva, at least if additional olfactory stimuli indicate that the object may be a predator. Over the years, we noted about 10 cases of a Pachyoliva turning immobile upon contacting an Agaronia laterally (three of the incidents that we were able to film are documented in Videos 1–3 in the Supplement; Ulate Gómez et al., 2023). None of these encounters resulted in an attack directed at the Pachyoliva, suggesting a significant defensive efficiency of this predator-induced immobility response.
The definition of freezing cited above – immobility used to reduce the risk of predator detection or tracking – certainly is met in cases such as those in Videos 1 and 2 in the Supplement. The situation is less clear where olfactory cues appear to betray the presence of the prey. In the example presented as Fig. 1b–e and Video 3 in the Supplement, the misguided attack strike leaves little doubt that the predator is aware of the prey's presence, although it fails to localize it correctly. While implications of this distinction – freezing to prevent detection of presence vs. freezing to prevent precise localization – for our interpretations of the behaviour of Pachyoliva and Agaronia as well as of other species remain to be explored, it is evident that Pachyoliva performs post-contact freezing. Freezing therefore cannot always be distinguished from “post-contact immobility” (i.e. thanatosis; Sendova-Franks et al., 2020) by simply noting when physical contact occurs. Alternative criteria may have to be employed depending on the sensory repertoire of the species involved.
It seems almost trivial that defensive responses of prey species may be evoked by a variety of sensory inputs (e.g. Humphreys and Ruxton, 2019, pp. 578–579). However, as John Endler remarked some 40 years ago, in the behavioural ecology of predator–prey interactions “most work has been done with visual signals, and there is a remarkable neglect of defenses operating in hearing, olfaction, chemoreception, lateral line, and electrical sensory modes” (Endler, 1986, p. 112). One may doubt that the situation has improved much; the case discussed here suggests that mechano-perception should be added to the list of understudied sensory modes.
Data used in creating Fig. 2 are available online at https://doi.org/10.5281/zenodo.10050551 (Ulate Gómez et al., 2023).
Videos 1–5 are available online at https://doi.org/10.5281/zenodo.10050551 (Ulate Gómez et al., 2023).
WSP conceived of and supervised this study. The field experiment was designed and conducted by TNUG, AEW, and WSP. WSP analysed data and wrote the draft, which was edited and finally approved by all authors.
The contact author has declared that none of the authors has any competing interests.
Publisher’s note: Copernicus Publications remains neutral with regard to jurisdictional claims made in the text, published maps, institutional affiliations, or any other geographical representation in this paper. While Copernicus Publications makes every effort to include appropriate place names, the final responsibility lies with the authors.
We thank Carlos E. Chacon Vargas for initiating the collaboration between the North American and Costa Rican authors.
This paper was edited by Matthias Foellmer and reviewed by Aaren Freeman and Takahisa Miyatake.
Asakura, M., Matsumura, K., Ishihara, R., and Miyatake, T.: Freezing or death-feigning? Beetles selected for long death feigning showed different tactics against different predators, Ecol. Evol., 12, e8533, https://doi.org/10.1002/ece3.8533, 2022.
Carli, G. and Farabollini, F.: Synthesis of defense response characteristics, Prog. Brain Res., 271, 331–337, https://doi.org/10.1016/bs.pbr.2022.02.013, 2022.
Caro, T.: Antipredator defenses in birds and mammals, University of Chicago Press, Chicago, ISBN 0-226-09436-7, 2005.
Chelini, M. C., Willemart, R. H., and Hebets, E. A.: Costs and benefits of freezing behaviour in the harvestman Eumesossoma roeweri (Arachnida, Opiliones), Behav. Process., 82, 153–159, https://doi.org/10.1016/j.beproc.2009.06.001, 2009.
Cooper, W. E. and Blumstein, D. T. (Eds.): Escaping from predators, Cambridge University Press, Cambridge, ISBN 1-107-63063-0, 2018.
Cyrus, A. Z., Rupert, S. D., Silva, A. S., Graf, M., Rappaport, J. C., Paladino, F. V., and Peters, W. S.: The behavioural and sensory ecology of Agaronia propatula (Caenogastropoda: Olividae), a swash-surfing predator on sandy beaches of the panamic faunal province, J. Mollus. Stud., 78, 235–245, https://doi.org/10.1093/mollus/eys006, 2012.
De Franceschi, G., Vivattanasarn, T., Saleem, A. B., and Solomon, S. G.: Vision guides selection of freeze or flight defense strategies in mice, Curr. Biol., 26, 2150–2154, https://doi.org/10.1016/j.cub.2016.06.006, 2016.
Edmunds, M.: Defence in animals, Longman, London, ISBN 0-582-44132-3, 1974.
Eilam, D.: Die hard: a blend of freezing and fleeing as a dynamic defense – implications for the control of defensive behavior, Neurosci. Biobehav. R., 29, 1181–1191, https://doi.org/10.1016/j.neubiorev.2005.03.027, 2005.
Endler, J. A.: Defense against predators, in: Predator-prey relationships, edited by: Feder, M. E. and Lauder, G. V., University of Chicago Press, Chicago, 109–134, ISBN 0-226-23945-4, 1986.
Endler, J. A.: Interactions between predators and prey, in: Behavioural ecology. An evolutionary approach, 3rd edn., edited by: Krebs, J. R. and Davies, N. B., Blackwell Scientific Publications, London, 169–196, ISBN 0-632-02702-9, 1991.
Honma, A.: The function of tonic immobility: review and prospects, in: Death-feigning in insects, edited by: Sakai, M., Springer Nature, Singapore, 23–37, https://doi.org/10.1007/978-981-33-6598-8_3, 2021.
Honma, A., Oku, S., and Nishida, T.: Adaptive significance of death feigning posture as a specialized inducible defence against gape-limited predators, P. R. Soc. B, 273, 1631–1636, https://doi.org/10.1098/rspb.2006.3501, 2006.
Humphreys, R. K. and Ruxton, G. D.: A review of thanatosis (death feigning) as an antipredator behaviour, Behav. Ecol. Sociobiol., 72, 22, https://doi.org/10.1007/s00265-017-2436-8, 2018.
Humphreys, R. K. and Ruxton, G. D.: Dropping to escape: a review of an under-appreciated antipredator defense, Biol. Rev., 94, 575–589, https://doi.org/10.1111/brv.12466, 2019.
Kantor, Y. I. and Tursch, B.: Feeding, in: Oliva shells. The genus Oliva and the species problem, edited by: Tursch, B. and Greifeneder, D., L'Informatore Piceno, Ancona, Italy, 209–216, ISBN 8607-17-9, 2001.
Marcus, E. and Marcus, E.: Studies on Olividae, Boletim da Faculdade de Filosofia, Ciências e Letras, Universidade de São Paulo. Zoologia, 22, 99–188, https://doi.org/10.11606/issn.2526-3382.bffclzoologia.1959.120324, 1959.
Misslin, R.: The defense system of fear: behavior and neurocircuitry, Neurophysiol. Clin., 33, 55–66, https://doi.org/10.1016/S0987-7053(03)00009-1, 2003.
Miyatake, T., Katayama, K., Takeda, Y., Nakashima, A., Sugita, A., and Mizumoto, M.: Is death-feigning adaptive? Heritable variation in fitness difference of death-feigning behaviour, P. R. Soc. B, 271, 2293–2296, https://doi.org/10.1098/rspb.2004.2858, 2004.
Pastorino, G. and Peters, W. S.: Radula morphology confirms the distinct status of the suspension-feeding Pachyoliva Olsson, 1956, among Olivellinae (Caenogastropoda: Olividae), Nautilus, 137, 1–6, 2023.
Peters, W. S.: Olivas nas Costas do Pacífico da América Central / Olive Shells on Pacific Coasts of Central America, Version v1, Zenodo [video], https://doi.org/10.5281/zenodo.7311382, 2022.
Peters, W. S.: “Freezing” in Pachyoliva semistriata (Caenogastropoda: Olividae) is induced olfactorily by its main predator and differs from unspecific avoidance behaviour, Arch. Molluskenkd., 152, 25–33, https://doi.org/10.1127/arch.moll/152/025-033, 2023.
Robinson, N. J. and Peters, W. S.: Complexity of the prey spectrum of Agaronia propatula (Caenogastropoda: Olividae), a dominant predator in sandy beach ecosystems of Pacific Central America, PeerJ, 6, e4714, https://doi.org/10.7717/peerj.4714, 2018.
Rogers, S. M. and Simpson, S. J.: Thanatosis, Curr. Biol., 24, R1031–R1033, https://doi.org/10.1016/j.cub.2014.08.051, 2014.
Ruxton, G.: Grasshoppers don't play possum, Nature, 440, 880, https://doi.org/10.1038/440880a, 2006.
Sakai, M.: Freezing and tonic immobility: their definitions and naming, in: Death-feigning in insects, edited by: Sakai, M., Springer Nature, Singapore, 1–14, https://doi.org/10.1007/978-981-33-6598-8_1, 2021.
Sendova-Franks, A. B, Worley, A., and Franks, N. R.: Post-contact immobility and half-lives that save lives, P. R. Soc. B, 287, 20200881, https://doi.org/10.1098/rspb.2020.0881, 2020.
Troost, A. I., Rupert, S. D., Cyrus, A. Z., Paladino, F. V., Dattilo, B. F., and Peters, W. S.: What can we learn from confusing Olivella columellaris and O. semistriata (Olivellidae, Gastropoda), two key species in panamic sandy beach ecosystems? Biota Neotrop., 12, 101–113, https://doi.org/10.1590/S1676-06032012000200011, 2012.
Ulate Gómez, T. N., Welch, A. E., and Peters, W. S.: Supplementary Videos and Data for “Problems of classifying predator-induced prey immobility: an unexpected case of post-contact freezing”, Zenodo [data set/video], https://doi.org/10.5281/zenodo.10050551, 2023.
Veelenturf, C. A. and Peters, W. S.: Size-dependent locomotory performance creates a behaviorally mediated prey size refuge in the marine snail Olivella semistriata: a study in the natural habitat, Curr. Zool., 66, 57–62, https://doi.org/10.1093/cz/zoz022, 2020.