the Creative Commons Attribution 4.0 License.
the Creative Commons Attribution 4.0 License.
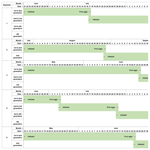
Emergence timing and voltinism of phantom midges, Chaoborus spp., in the UK
Robert J. G. Cockroft
W. Richard Jenkins
Anthony G. Irwin
Steve Norman
Kevin C. Brown
Phantom midges, Chaoborus spp. (Diptera: Chaoboridae), are an important taxon in environmental risk assessment of plant protection products due to the sensitivity of their larvae to insecticides. To aid modelling of population responses to xenobiotic exposure, information on emergence timing and voltinism is needed, but definitive evidence to support these parameters is lacking in the literature. We investigated emergence timing of overwintering Chaoborus larvae and the number of life cycles occurring per year in two separate, mesh-enclosed outdoor microcosm experiments in the spring and summer of 2017.
Emergence from overwintering larvae of a population of predominantly C. obscuripes (99.68 %) commenced on 13 April and peaked on 2 May. The majority of emergence was completed by 3 June. Emergence success ranged from 51.4 % to 66.2 %, indicating that for overwintered C. obscuripes larvae, adults emerged contemporaneously in spring, rather than sporadically over the course of spring and summer.
A population of C. crystallinus larvae produced up to four discrete generations over the spring and summer months (i.e. were confirmed to be multivoltine), with life cycle durations (egg-to-egg) ranging from 14 to 56 d. The differences in life cycle strategy observed in this study have implications for assessment of the capacity of populations of Chaoborus spp. to recover if there are localised impacts due to insecticide exposure or other stressors.
- Article
(513 KB) - Full-text XML
-
Supplement
(126 KB) - BibTeX
- EndNote
Phantom midges (Chaoborus spp.) are a focal taxon in the environmental risk assessment of some classes of plant protection products due to their high sensitivity to acute exposure and the complexity of their life histories (Dohmen et al., 2016), in addition to their importance as regulators of zooplankton populations (Janz et al., 2016) and ecosystem services such as provision of nutrient subsidies to riparian ecosystems (Parmar et al., 2022). The larval stages are sensitive to the effects of insecticides (Maund et al., 1998; Schroer et al., 2004; van Wijngaarden et al., 2009), and post-exposure population recovery can affect concomitant zooplankton recovery (Hanson et al., 2007). Therefore, C. crystallinus has been used in modelling to predict effects and recovery in aquatic invertebrates (Strauss et al., 2016). Life cycle duration, timing and numbers of generations per year are critical variables in modelling populations of aquatic insects (Mebane et al., 2020) and are therefore important for understanding potential recovery following localised extinctions due to chemical exposures (Dohmen et al., 2016; Gergs et al., 2016), in addition to verification of “no-observed-effect concentrations” from microcosm toxicity studies using estimation of minimum detectable difference (Brock et al., 2015; Duquesne et al., 2020).
Chaoboridae are holometabolous Diptera with pelagic larvae progressing through four instars, hereinafter L1–L4, preying on zooplankton, including other chaoborids. Habitat preferences for Europe's more common species are well documented (Parma, 1969a, b, 1971; Borkent, 1981; Arranz et al., 2015). Hatching duration ranges from 190–200 h at 10 ∘C and 37–50 h at 20 ∘C (Parma, 1969b). Conspecific chaoborid larvae have variable life spans (Parma, 1971) depending on environmental conditions and zooplankton community structure. The pupal stage for C. crystallinus can take 2–4 d at 20 ∘C, 10–13 d at 10 ∘C and 30 d at 5 ∘C. In central Europe, there is a pronounced emergence period between April and May with a second diffuse emergence from late July to October (Parma, 1971).
Life cycle strategies of Chaoborus spp. are not so well evidenced in the literature. The presence of larvae throughout the year has prompted assumptions that Chaoborus are univoltine in temperate conditions. C. crystallinus had been considered univoltine or bivoltine (Parma, 1969b, 1971). Berendonk and Spitze (2006) state that C. crystallinus is typically univoltine in central Europe, although it may be bivoltine in exceptionally hot summers, while Roessink et al. (2005) refer to C. obscuripes as multivoltine. However, no supporting empirical evidence is cited in either case. More recently, some empirical evidence for multivoltinism has been reported. Data collected from 19 microcosm studies spanning 14 years at the Technical University of Munich, Germany, showed that L1s and L2s were present from mid-April to early October and L3s from early May to October or November (Janz et al., 2016). Larvae overwintered as L4s, which were present throughout the year. Pupae were found from early April to late August. Ovipositing peaks were assumed to indicate that there were three generations of C. crystallinus: one ovipositing in late April/early May, the second in June and the third in late July/early August (Janz et al., 2016). This study refines that approach, tracking development of successive generations by surrounding microcosms with mesh enclosures to exclude the confounding factor of immigrant oviposition and to reduce the reliance on assumptions to delineate completed life cycles.
The study was intended to evaluate (1) whether emergence of adults from the overwintering cohort of L4 larvae would be protracted over the entire summer season and (2) whether more than two discrete generations could be produced per year (i.e. that pond-dwelling Chaoborus spp. are multivoltine).
Two experiments were conducted at AgroChemex Environmental Ltd., Aldhams Farm Research Station, Essex, UK (grid reference TM09943048; grid references are according to the Ordnance Survey National Grid), in 2017, the first to investigate the emergence timing of Chaoborus adults from an overwintered cohort of L4s and the second to determine how many discrete generations per year could occur.
Established February–March 2017, the microcosms used in both experiments were of identical design, comprising circular polypropylene tanks 0.8 m in diameter and 0.6 m deep, sunk into turfed ground to a depth of 0.5 m. Washed sharp sand (20 L) was added, and each microcosm was filled to a depth of 0.5 m with freshly drawn borehole water. Approximately 10 L of water-saturated lake sediment was added, after sieving to remove invertebrates. Each unit was covered with 1 mm2 aperture mesh to exclude immigrating aquatic flies. Oxygenating macrophytes (Elodea canadensis, sourced from Labcorp, Eye, Suffolk, UK) were rinsed thoroughly to remove invertebrates and loosely planted in each microcosm, occupying approximately 25 % of the sediment surface. Zooplankton and benthic detritivores, sourced from a natural pond on site, were added to each microcosm.
Active microcosms were monitored weekly for physicochemical water quality parameters (temperature, pH, dissolved oxygen and conductivity) using a Hach HQ40D multimeter. Water temperatures in one unit per experiment were monitored using calibrated data loggers, with readings every 30 min, and in an unused, unenclosed microcosm from June onwards to compare temperatures in enclosed and unenclosed systems. Climatic conditions were monitored continuously through the experimental season using a Vantage Pro2™ weather station (Davis Instruments Corporation, Hayward, CA, USA) positioned approximately 115 m from the microcosm array (grid reference TM10053044).
2.1 Emergence timing
Chaoborus populations were seeded on 22 March 2017 by adding nominally 500 L4s, obtained from an untreated reservoir at Labcorp, Huntingdon, UK, to each of four replicate microcosms. Prior to seeding, the initial mesh coverings were replaced with pop-up frames and insect-proof mesh (Popadome, Harrod Horticultural, Lowestoft, UK) (“enclosure(s)”). Emergence of adult Chaoborus spp. was monitored daily by collecting insects seen within the enclosure using a vacuum sampler. Specimens were preserved for identification in 70 % alcohol. The water surface was inspected daily for oviposition. Eggs were removed immediately to prevent recruitment of F1 larvae.
2.2 Voltinism in Chaoborus
This experiment comprised six replicates each of four individual microcosms, established between February and March 2017. Four additional microcosms were prepared to provide the initial eggs. These were covered with enclosures, and each was seeded with approximately 500 Chaoborus L4s from the same source as the emergence experiment.
During adult emergence, these microcosms were monitored regularly for the presence of eggs, which are laid as rafts on the water surface. Eggs were transferred to the first microcosm in each replicate set, and in each seeded microcosm, the initial mesh covering was replaced with an enclosure. Seeded microcosms were monitored for the presence of larvae and pupae, using a 19 cm diameter white disc, attached to a rod, for contrast. Adults were assessed by inspection of the enclosure mesh.
Adults were left within the enclosure to reproduce. Resultant eggs were then transferred to the second microcosm of each replicate set to initiate the subsequent generation. Each established microcosm was inspected three times per week. At the end of September, monitoring frequency was reduced to once weekly. The presence of each life stage of Chaoborus was recorded in each active microcosm. Where larvae were present, the approximate instar (estimated by eye) and the presence of pupae were recorded to ensure that critical development stages were not missed. Once emergence had been observed, at each subsequent assessment, the water surface was inspected for eggs. The observation of the first oviposition was recorded, and those eggs were used to start the next sequential unit within the replicate. Subsequently, additional eggs laid within the active units were also transferred to supplement the next generation, until viable L1s were established.
Monitoring the life cycle was repeated for the subsequent generations, as applicable. The date from the first oviposition in each generation was used to estimate the egg-to-egg life cycle duration. On three occasions, once in July and twice in October, samples of late-instar larvae were preserved in 70 % alcohol for taxonomic identification. In the July sampling, only three microcosms contained larvae sufficiently developed for identification. Ten larvae were sampled from each. In October, all larvae that could be captured were preserved, up to a maximum of approximately 30 per microcosm. Larvae were identified to the species level using a Leica S8 APO stereomicroscope (×10–×80) with reference to dichotomous keys.
Statistical analyses, where applicable, were carried out using IBM SPSS Statistics Version 28.0. For both experiments, the normality of distribution and homogeneity of variance of the four water quality parameters were checked using Shapiro–Wilk and Levene's tests, respectively. Since the datasets for some water quality parameters were not normally distributed, replicates were compared (within each experiment) using Kruskal–Wallis nonparametric tests.
3.1 Emergence timing
Emergence of adult Chaoborus from overwintered larvae commenced on 13 April and peaked on 2 May. Most emergence was completed by early June (the latest that emergence was recorded in all four replicates was 3 June). Of the 2027 L4s introduced, the mean emergence success was 60.9 %, ranging from 51.4 % to 66.2 %. Of the 1228 adults, all but 4 were C. obscuripes. The numbers of emerged male and female C. obscuripes in each replicate are shown in Fig. 1.
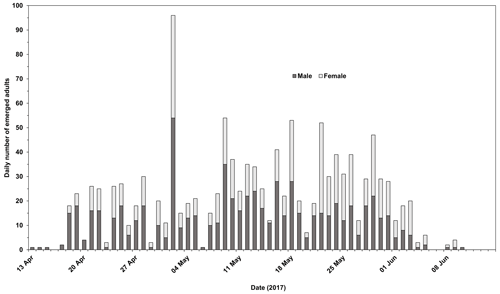
Figure 1Emergence timing of male and female Chaoborus obscuripes. Males appeared to emerge slightly earlier than females.
Three specimens of C. crystallinus were found, the first on 24 April and one each on 1 and 5 September, respectively. A single adult sampled on 22 May was only identifiable to genus level. Therefore, of the emerged adults, 99.68 % were C. obscuripes and 0.24 % were C. crystallinus.
Water temperature, dissolved oxygen, pH and conductivity did not vary significantly between microcosms. Summary statistics and nonparametric comparison results are given in Table S1 in the Supplement, and the raw data are available in the Supplement.
3.2 Voltinism
The development of Chaoborus from the initial eggs introduced is presented in Fig. 2. Eggs seeded into the first microcosms of Replicates 1, 2, 4 and 5 failed to establish at the first attempt, and Replicates 1, 2 and 5 were reinitiated as fresh eggs became available. Reinitiated Replicates 1 and 5 both progressed through to fourth generations. These two replicates contained C. obscuripes and C. crystallinus in their respective first units although the larvae sampled from Units 2–4 were all C. crystallinus. Reinitiation of Replicate 2 produced only two generations, which, when sampled in October, were found to comprise only C. crystallinus.
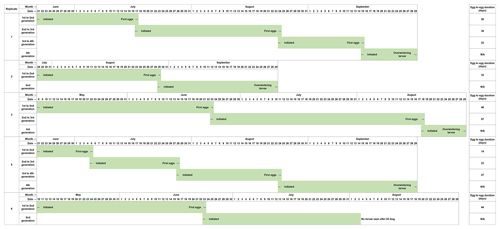
Figure 2Development of C. crystallinus from introduced egg rafts in each microcosm that sustained populations in the life cycle study. Replicate 4 could not be reinitiated with egg rafts, as none were available at a suitable time. Monitoring of this unit showed that no larvae were present at any time, confirming the effectiveness of the systems in preventing colonisation by Chaoborus spp. from other sources.
Populations of Chaoborus in Replicates 3 and 5 progressed through to third and fourth generations, respectively. Larvae sampled from Unit 1 in July were all C. obscuripes, although when re-sampled in October, both C. obscuripes and C. crystallinus were found. Generations 2 and 3, both sampled in October, comprised only C. crystallinus. Replicate 6 also did not require reinitiation, but Unit 2 did not establish successfully and its population died out. Six late-instar larvae remained in Unit 1 by the October sampling, all C. obscuripes.
Egg-to-egg times for C. crystallinus ranged from 14 to 56 d. The shortest generation time occurred when the water temperature was at its highest in late June and early July. However, the longest observed development time also spanned this period, so other environmental parameters or variables such as prey density are clearly involved in determining the Chaoborus development rate.
Water temperature and dissolved oxygen, recorded weekly during the study, did not differ significantly between replicates. However, there were statistically significant between-replicate differences in pH and conductivity (Table S2).
There was a marked shift in dominance from C. obscuripes in the first generations to C. crystallinus in the subsequent generations, although populations of C. obscuripes persisted in the first units of four out of the five productive replicates (Table 1).
The emergence of adult C. obscuripes originating from the pupation of post-overwintering L4s took place over a clearly defined period between mid-April and early June. Males appeared to emerge slightly earlier than females (Fig. 1). In the first week of emergence, 42 males were recorded compared with 8 females. All except four of the emergent adults were C. obscuripes, which was consistent with the populations of Chaoborus known to inhabit the source pond in previous years.
The voltinism study showed that C. crystallinus produced up to four discrete generations within the experimental period. Since both replicates producing four generations were reinitiated several weeks after the season's oviposition commenced, one more generation may have been possible. There were some differences in development times, voltinism or reproductive success between species, since no C. obscuripes were sampled from second or later generations, despite their presence in the first generations and persistence until the end of the experiment. Despite the substantial overlap in the natural distributions of the two species (Borkent, 1981), it is possible that elements of the study conditions favoured C. crystallinus. Interspecific variation in larval tolerance to UV radiation has been reported (Nagiller and Sommaruga, 2009), while UV radiation has also been demonstrated to reduce the kairomone-induced defences against predation by C. obscuripes in the cladoceran Daphnia pulex (Sterr and Sommaruga, 2008), mediated by the effects of UV on the chemical integrity of kairomones released by the predator. Additionally, feeding in C. obscuripes larvae has been shown to be adversely affected by increasing pCO2 (Kowalewska et al., 2020), so it seems possible that night-time releases of CO2 from macrophytes, which were not measured but were likely to have been substantial given the daytime extremes of dissolved oxygen (see the Supplement), may have impaired C. obscuripes reproduction in this study. However, since all reinitiated replicates contained only C. crystallinus, this could indicate that all mating and ovipositing in C. obscuripes were completed by the time the eggs used for reinitiating were harvested. If so, this would indicate that C. obscuripes is truly univoltine, a view supported by the evidence from the emergence study described here, in which no C. obscuripes larvae from the overwintering initial cohort remained after mid-June, and from the life cycle study, in which larvae of C. obscuripes were found in the first units of some replicates, even though the mesh enclosures remained in place, preventing immigrating adults from ovipositing.
The time for development from egg to egg ranged from 14 to 56 d with a mean of 32.8 d. As significant causal links between environmental conditions and development time were limited, it seems most likely that varying prey quality or availability contributed to the wide range of development times.
Limitations and extensions
We made no attempt to quantify or characterise the zooplankton prey populations as prey availability was outside the scope of the study. As a variable likely to be influential in determining the rate of ontogeny, this would be valuable to integrate into further work.
We could not present any evidence for multivoltinism in C. obscuripes, despite their dominance in the initial cohort. As it is not possible to identify larvae to species level non-destructively, we could not initiate monospecific populations, so the factor of interspecific competition could not be controlled for.
The qualitative nature of the study meant that we were unable to support our findings with statistical analysis of organism abundance. However, to replicate the procedure in subsequent years or in other locations could elucidate the influence of environmental conditions on voltinism. Similarly, interspecific variation in voltinism could be more conclusively demonstrated by robust taxonomic identification of the initiating organisms.
Remodelling of this experimental design to include treatment with a xenobiotic of known toxicity to C. crystallinus and C. obscuripes could more conclusively elucidate differences in recovery potential between the two species.
This study confirmed that under temperate conditions, emergence of overwintering C. obscuripes larvae is not protracted over an entire summer and that C. crystallinus is multivoltine. This may indicate a higher capability for populations to recover from localised extinction. Recovery in C. obscuripes has been documented previously (e.g. Van Wijngaarden et al., 2006; Brock et al., 2010). The former authors, however, showed that recovery in C. obscuripes was much more pronounced after a spring application than in late summer, which appears to have been supported by the finding that emergence in overwintering L4s of this species is largely complete by mid-summer. Recovery, therefore, appears to be enhanced during periods of emergence and immigration from unaffected neighbouring sources, which suggests that C. crystallinus metapopulations may be better adapted to withstand localised extinctions than C. obscuripes.
The number of life cycles in C. crystallinus was positively correlated with daily minimum (but not mean or maximum) air temperature and negatively correlated with the UV index and solar radiation. These three parameters in combination explained a significant portion of the variation in voltinism, but none, taken in isolation, made a significant contribution. Therefore, it is possible that variation in these parameters acted only indirectly on the performance of the C. crystallinus populations, possibly by affecting food web interactions. For example, Tseng et al. (2021) showed that temperature has a significant effect on synthesis of important fatty acids at the primary producer level, which could have a concomitant influence on nutritional quality for secondary and tertiary consumers. Sentis et al. (2017) found a significant reduction in prey handling time (i.e. more effective feeding) in C. obscuripes with an increase in water temperature from 16 to 20 ∘C. Lastly, Lindholm et al. (2016) found the congener C. nyblaei to need attenuation of UV by substantial discolouration of the water in order to act as a significant top-down predator.
The life cycle duration (in this study ranging from 14–56 d for C. crystallinus) has implications for intraspecific variation in the sensitivity of the larvae to xenobiotics. For example, it could be hypothesised that the longer the life cycle duration, the longer each larva spends in each development stage, and, in particular, long durations of early instars with smaller body sizes may render them more sensitive to toxicants (Gergs et al., 2015).
Supporting water quality data and values required to plot Fig. 1 are given in the Supplement and at https://doi.org/10.5281/zenodo.7293656 (Cockroft et al., 2022).
The supplement related to this article is available online at: https://doi.org/10.5194/we-22-101-2022-supplement.
RJGC, WRJ and SN designed the experiments, and RJGC carried them out. AGI advised on test organism behaviour at the study design stage and carried out all taxonomic identification. KCB prepared Fig. 1 and an earlier version of the manuscript with contributions from all co-authors. RJGC prepared this version of the paper with contributions from all co-authors.
The contact author has declared that none of the authors has any competing interests.
The funding organisation did not influence the experimental design, conduct or reporting stages, or the conclusions drawn from the study findings.
Publisher’s note: Copernicus Publications remains neutral with regard to jurisdictional claims in published maps and institutional affiliations.
The authors gratefully acknowledge the constructive input we received from the subject editor and the anonymous reviewers.
The work was funded by ADAMA Makhteshim Limited, although the authors retained full control over the experimental design, conduct and interpretation.
This paper was edited by Matthias Foellmer and reviewed by two anonymous referees.
Arranz, I., Sala, J., Gascón, S., Ruhí, A., Quintana, X. D., Amoedo, J., and Martinoy, M.: Contribution to the knowledge of the distribution of Chaoborus species (Diptera: Chaoboridae) in the NE Iberian Peninsula, with notes on the spatial and temporal segregation among them, Limnetica, 34, 57–68, https://doi.org/10.23818/limn.34.05, 2015.
Berendonk, T. and Spitze, K.: Gene flow within and between regions: The population genetic structure of the phantom midge Chaoborus crystallinus (Diptera: Chaoboridae), Limnologica., 36, 147–154, 2006.
Borkent, A.: The distribution and habitat preferences of the Chaoboridae (Culicomorpha: Diptera) of the Holarctic Region, Can. J. Zool., 59, 122–133, https://doi.org/10.1139/z81-019, 1981.
Brock, T. C. M., Belgers, J. D. M., Roessink, I., Cuppen, J. G. M., and Maund, S. J.: Macroinvertebrate responses to insecticide application between sprayed and adjacent nonsprayed ditch sections of different sizes, Environ. Toxicol. Chem., 29, 1994–2008, https://doi.org/10.1002/etc.238, 2010.
Brock, T. C. M., Hammers-Wirtz, M., Hommen, U., Preuss, T. G., Ratte, H.-T., Roessink, I., Strauss, T., and Van den Brink, P. J.: The minimum detectable difference (MDD) and the interpretation of treatment-related effects of pesticides in experimental ecosystems, Environ. Sci. Pollut. R., 22, 1160–1174, https://doi.org/10.1007/s11356-014-3398-2, 2015.
Cockroft, R. J. G., Jenkins, W. R., Irwin, A. G., Norman, S., and Brown, K. C.: Emergence timing and voltinism of phantom midges, Chaoborus spp., in the UK, Zenodo [data set], https://doi.org/10.5281/zenodo.7293656, 2022.
Dohmen, G. P., Preuss, T. G., Hamer, M., Galic, N., Strauss, T., van den Brink, P. J., De Laender, F., and Bopp, S.: Population-level effects and recovery of aquatic invertebrates after multiple applications of an insecticide, Integr. Environ. Asses., 12, 67–81, https://doi.org/10.1002/ieam.1676, 2016.
Duquesne, S., Alalouni, U., Gräff, T., Frische, T., Pieper, S., Egerer, S., Gergs, R., and Wogram, J.: Better define beta–optimizing MDD (minimum detectable difference) when interpreting treatment-related effects of pesticides in semi-field and field studies, Environ. Sci. Pollut. R., 27, 8814–8821, https://doi.org/10.1007/s11356-020-07761-0, 2020.
Gergs, A., Kulkarni, D., and Preuss, T. G.: Body size-dependent toxicokinetics and toxicodynamics could explain intra- and interspecies variability in sensitivity, Environ. Pollut., 206, 449–455, https://doi.org/10.1016/j.envpol.2015.07.045, 2015.
Gergs, A., Classen, S., Strauss, T., Ottermanns, R., Brock, T. C. M., Ratte, H. T., Hommen, U., and Preuss, T. G.: Ecological Recovery Potential of Freshwater Organisms: Consequences for Environmental Risk Assessment of Chemicals, in: Reviews of Environmental Contamination and Toxicology, edited by: de Voogt, P., Springer International Publishing, Cham, 236, 259–294, https://doi.org/10.1007/978-3-319-20013-2_5, 2016.
Hanson, M. L., Graham, D. W., Babin, E., Azam, D., Coutellec, M.-A., Knapp, C. W., Lagadic, L., and Caquet, T.: Influence of isolation on the recovery of pond mesocosms from the application of an insecticide. I. Study design and planktonic community responses, Environ. Toxicol. Chem., 26, 1265–1279, https://doi.org/10.1897/06-248R.1, 2007.
Janz, P., Weltje, L., Ebke, K. P., and Dawo, U.: Temporal population dynamics of the phantom midge Chaoborus crystallinus and its influence on the zooplankton community, Hydrobiologia, 770, 273–287, https://doi.org/10.1007/s10750-015-2602-9, 2016.
Kowalewska, A. A., Krebs, N., Tollrian, R., and Weiss, L. C.: Elevated pCO2 affects behavioural patterns and mechano-sensation in predatory phantom midge larvae Chaoborus obscuripes, Sci. Rep., 10, 1800, https://doi.org/10.1038/s41598-020-58763-4, 2020.
Lindholm, M., Wolf, R., Finstad, A., and Hessen, D. O.: Water browning mediates predatory decimation of the Arctic fairy shrimp Branchinecta paludosa, Freshwater Biol., 61, 340–347, https://doi.org/10.1111/fwb.12712, 2016.
Maund, S. J., Hamer, M. J., Warinton, J. S., and Kedwards, T. J.: Aquatic ecotoxicology of the pyrethroid insecticide lambda-cyhalothrin: considerations for higher-tier aquatic risk assessment, Pestic. Sci., 54, 408–417, https://doi.org/10.1002/(SICI)1096-9063(199812)54:4<408::AID-PS843>3.0.CO;2-T, 1998.
Mebane, C. A., Schmidt, T. S., Miller, J. L., and Balistrieri, L. S.: Bioaccumulation and Toxicity of Cadmium, Copper, Nickel, and Zinc and Their Mixtures to Aquatic Insect Communities, Environ. Toxicol. Chem., 39, 812–833, https://doi.org/10.1002/etc.4663, 2020.
Nagiller, K. and Sommaruga, R.: Differential tolerance of UV radiation between Chaoborus species and role of photoprotective compounds, J. Plankton Res., 31, 503–513, https://doi.org/10.1093/plankt/fbn133, 2009.
Parma, S.: Notes on the larval taxonomy, ecology, and distribution of the Dutch Chaoborus species (Diptera, Chaoboridae), Beaufortia, 17, 21–50, 1969a.
Parma, S.: The life cycle of Chaoborus crystallinus (DeGeer) (Diptera, Chaoboridae) in a Dutch pond, Internationale Vereinigung für Theoretische und Angewandte Limnologie: Verhandlungen, 17, 888–894, https://doi.org/10.1080/03680770.1968.11895933, 1969b.
Parma, S.: Chaoborus flavicans (Meigen) (Diptera:Chaoboridae): An autoecological study, PhD thesis, University of Groningen, Groningen, 1971.
Parmar, T. P., Kindinger, A. L., Mathieu-Resuge, M., Twining, C. W., Shipley, J. R., Kainz, M. J., and Martin-Creuzburg, D.: Fatty acid composition differs between emergent aquatic and terrestrial insects – A detailed single system approach, Frontiers in Ecology and Evolution, 10, 952292, https://doi.org/10.3389/fevo.2022.952292, 2022.
Roessink, I., Arts, G. H. P., Belgers, J. D. M., Bransen, F., Maund, S. J., and Brock, T. C. M.: Effects of lambda-cyhalothrin in two ditch microcosm systems of different trophic status, Environ. Toxicol. Chem., 24, 1684–1696, https://doi.org/10.1897/04-130R.1, 2005.
Schroer, A. F. W., Belgers, J. D. M., Brock, C. M., Matser, A. M., Maund, S. J., and Van den Brink, P. J.: Comparison of Laboratory Single Species and Field Population-Level Effects of the Pyrethroid Insecticide λ-Cyhalothrin on Freshwater Invertebrates, Arch. Environ. Con. Tox., 46, 324–335, https://doi.org/10.1007/s00244-003-2315-3, 2004.
Sentis, A., Gémard, C., Jaugeon, B., and Boukal, D. S.: Predator diversity and environmental change modify the strengths of trophic and nontrophic interactions, Glob. Change Biol., 23, 2629–2640, https://doi.org/10.1111/gcb.13560, 2017.
Sterr, B. and Sommaruga, R.: Does ultraviolet radiation alter kairomones? An experimental test with Chaoborus obscuripes and Daphnia pulex, J. Plankton Res., 30, 1343–1350, https://doi.org/10.1093/plankt/fbn087, 2008.
Strauss, T., Kulkarni, D., Preuss, T. G., and Hammers-Wirtz, M.: The secret lives of cannibals: Modelling density-dependent processes that regulate population dynamics in Chaoborus crystallinus, Ecol. Model., 321, 84–97, https://doi.org/10.1016/j.ecolmodel.2015.11.004, 2016.
Tseng, M., Di Filippo, C. M., Fung, M., Kim, J. O., Forster, I. P., and Zhou, Y.: Cascading effects of algal warming in a freshwater community, Funct. Ecol., 35, 920–929, https://doi.org/10.1111/1365-2435.13752, 2021.
Van Wijngaarden, R. P. A., Brock, T. C. M., van den Brink, P. J., Gylstra, R., and Maund, S. J.: Ecological Effects of Spring and Late Summer Applications of Lambda-Cyhalothrin on Freshwater Microcosms, Arch. Environ. Con. Tox., 50, 220–239, https://doi.org/10.1007/s00244-004-0249-z, 2006.
Van Wijngaarden, R. P. A., Barber, I., and Brock, T. C. M.: Effects of the pyrethroid insecticide gamma-cyhalothrin on aquatic invertebrates in laboratory and outdoor microcosm tests, Ecotoxicology, 18, 211–224, https://doi.org/10.1007/s10646-008-0274-1, 2009.