the Creative Commons Attribution 4.0 License.
the Creative Commons Attribution 4.0 License.
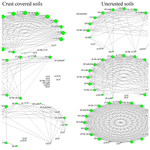
Spatial heterogeneity of Cladonia rangiformis and Erica spp. induces variable effects on soil microbial communities which are most robust in bare-soil microhabitats
Theofilos Dostos
Pantelitsa D. Kapagianni
Nikolaos Monokrousos
George P. Stamou
Efimia M. Papatheodorou
Biological soil crust (BSC) is an essential part of arid and semiarid regions; however information concerning the spatial heterogeneity of the interactions between crust, plants and soil microbes is limited. Sampling was confined to an area either covered with Erica spp. shrubs interspaced by crust cover (Cladonia rangiformis) or uncovered by crust. Along a straight line of 100 cm from the base of the shrubs towards their periphery and at successive distances of 20 cm, soil samples were collected once. The biomass, the composition and the network of interactions among the members of the soil microbial communities as well as the activity of soil enzymes involved in the C, N and P cycle were estimated. Crust coverage exerted a significant allelopathic negative effect on soil microbial biomasses due to the leaching of lichen's antimicrobial substances. In contrast, the crust effect on enzyme activity was positive, probably due to the amelioration of soil abiotic conditions. The distance from the base of Erica spp. affected the total microbial and bacterial biomass, with lower values at the base of the shrubs. The composition of microbial communities between the different sampling points exhibited significant dissimilarities. Network analysis revealed that in uncrusted soils the most connected microbial network was at samples collected from a distance of >60 cm (bare soils), while in crusted soils this was at samples collected at the base of shrubs. We concluded that microbial biomass showed limited response to spatial heterogeneity, while the composition and the topology of interactions among the microbial members reflected a heterogeneous soil environment existing on a small spatial scale in Mediterranean areas. The microbial community in bare soil appeared to be the most robust against future disturbances.
- Article
(2476 KB) - Full-text XML
-
Supplement
(658 KB) - BibTeX
- EndNote
Biological soil crust (BSC) is a complex microbial association composed of microorganisms, soil particles and extracellular polymeric substances. For the arid regions of the planet, it is estimated that biocrust covers up to 45 % of the soil surface, while for the Mediterranean region the percentage of coverage is almost 70 % (Steven et al., 2014). However, while BSCs are by definition found in arid and semiarid areas, this being their key component, they are also known as inhabitants of mesic ecosystems (Bowker, 2010).
Biocrusts are highly complex systems where the abiotic environment interacts with local soil organisms, including cyanobacteria, algae, lichens and mosses, bacteria, fungi, and various types of microfauna, inducing a series of geomorphological features, such as soil stabilization (Maier et al., 2018), increased corrosion resistance (Bu et al., 2015) and formation of a rough dust-trapping texture (Williams et al., 2012). In addition, crusts have an effect on the soil hydrological characteristics by altering water infiltration (Kidron et al., 2020), runoff intensity (Rodriguez-Caballiero et al., 2012) and evaporation rate (Kidron et al., 2020). Finally, crusts alter the soil surface temperature (Couradeau et al., 2016) and soil water content (Bu et al., 2015).
Given the environmental hardness predominating in arid and semiarid environments, the interaction of crusts with the vascular plants is vital for plants' survival (Bainbridge, 2012). Vegetation has an effect on biocrust formation by altering various microclimatic and territorial properties (Zhang et al., 2016). The type of plants, their density and the size of the canopy affect parameters such as the level of radiation reaching the soil, the quality of the litter produced and the soil temperature through the layer formed by the fallen leaves. Further plants interact with the biocrusts through the activity of their root system (Zhang et al., 2016). In crusted areas enhanced porosity and increased high soil humidity are recorded beneath plants (Menon et al., 2011). For its part, biological soil crust increases soil fertility and generally has a positive effect on soil quality, a fact that is reflected in the increase in soil enzyme activity underneath the crust (Liu et al., 2014). Especially in arid areas, where nutrient concentration is low, BSC presence is associated with increased levels of soil organic matter and available nitrogen through the fixation of atmospheric nitrogen (Weber et al. 2015), thus promoting the growth of vascular plants (Havrilla et al., 2019). Apart from increasing nutrients, the organisms of BSCs secrete exopolysaccharides especially at the initial stage of their development (Cania et al., 2020) and contain lipids, which act as energy reserves, contributing further to the development of an important energy source for soil biota (Ngosong et al., 2020). However, although in most cases the effects of biocrusts on soil properties were positive, Papatheodorou et al. (2020) mentioned that the abundance of soil microbes in areas covered by Cladonia rangiformis decreased after a rainy event. Similarly, Delgado-Baquerizo et al. (2015) found contrasting soil microbial abundances under the different biocrust-forming lichens. Specifically, the lichens Fulgensia subbracteata and Diploschistes diacapsis were negatively related to the abundance of soil bacteria.
Considering the importance of the BSCs' interplay with the local vegetation, in this study we focused on the effect of the interactions between the plant Erica spp. (a drought-tolerant shrub species predominating in Mediterranean areas) and the biocrust layer dominated by the lichen Cladonia rangiformis, on soil microbial biomass; community composition; and the activity of acid phosphatase, β-glucosidase, peroxidase, polyphenol oxidase, β-N-acetylglucosamine, leucine aminopeptidase and urease soil enzymes. In addition, in order to examine the effects of the spatial heterogeneity induced by crust's presence or absence combined with the plant presence on soil microbial attributes, the samples were collected along a straight line of 100 cm from the trunk of the shrubs towards their periphery in crusted and uncrusted soils. Soil habitats in Mediterranean areas are characterized as highly heterogeneous in time and space (fine grained mosaic; Stamou, 1998), where biotic and abiotic drivers affect the assemblages of soil communities across scales ranging from hectares to square centimeters (Wu et al., 2013). In this study, we hypothesized that the spatial heterogeneity will be reflected in quantitative microbial estimates. Higher microbial biomass and enzyme activity were expected at the base of the shrubs (0 to 20 cm from the trunk) in crust-covered areas where both biotic and abiotic conditions were favorable; high amounts of organic input (dead organic material and root exudates) are inserted into the soil, while crust cover acts as a buffer system for soil life against abiotic stress. Apart from quantitative estimates, we expected differences in the soil microbial community composition and the network of interactions among the microbial members even at distances of a few centimeters.
2.1 Experimental design and sampling
The sampling area was located in the Sithonia peninsula, at Halkidiki, northern Greece (40∘08′59.8`` N, 23∘54′56.2`` E). The climate of Sithonia is characterized by warm and dry summers and mild and moderately rainy winters. The area was dominated by Ericaceous shrubs interspaced with open areas covered by a well-developed biocrust layer, which consisted of the lichen Cladonia rangiformis (80 % cover) and mosses (Fig. S1 in the Supplement). The soil texture was sandy-loam (4 % clay, 20 % silt and 76 % sand), and the pH was 5.8 (soil : water ratio 1:2).
In early autumn (end of September), soil samples were collected at successive distances from the trunk of Erica spp. shrubs towards their periphery, at intervals of 20 cm along a straight line of 100 cm (six sampling points in total), in shrubs with crust-covered surrounding area (crusted shrub area) and those without crust (uncrusted shrub area). We sampled five crusted and five uncrusted shrubs that were distributed randomly in the wider area. For sampling, a soil auger of 5 cm diameter was used, and soil was collected from the upper 10 cm. The collected soil samples were placed in plastic bags after the removal of the crust in crust-covered samples, transferred to a laboratory, passed through a 2 mm sieve and stored at 4 ∘C until analysis.
2.2 Biochemical parameters
Soil water content (WC) was determined by a gravimetric method. Soil organic C was determined by a wet oxidation titration procedure using an acid dichromate system and soil organic nitrogen by the Kjeldahl method (Allen, 1974).
2.3 Enzyme activity
We assessed enzyme activity involved in C, P and N cycles. The enzymes b-glucosidase (BG), peroxidase (POD) and polyphenol oxidase (PPO) participate in the C cycle; BG hydrolyzes cellobiose to glucose (Knight and Dick, 2004), while POD and PPO catalyze the oxidation of recalcitrant aromatic compounds, such as lignin, into more readily available substrates (Cullen and Kersten, 1996). Acid phosphatase (AP) participates in the P cycle by hydrolyzing phosphomonoesters and in some cases phosphodiesters, releasing phosphate (Turner et al., 2002), while N-acetyl-glucosaminidase (NAG), leucine aminopeptidase (LAP) and urease (UR) are enzymes that participate in the N cycle. NAG degrades chitin (Felse and Panda, 1999), and LAP hydrolyzes leucine and other hydrophobic amino acids (Stursova et al., 2006), while UR decomposes urea into carbon dioxide and ammonium (Sinsabaugh et al., 2000). The activity of all enzymes was determined according to the methods described in detail by Kapagianni et al. (2020). The activity of AP, BG and NAG was expressed as µmol pNP g−1 soil h−1, LAP as µmol pNA g−1 soil h−1, PPO and POD as µmol L-DOPA g−1 soil h−1, and that of UR as µmol NH4+ g−1 soil h−1.
2.4 Determination of microbial biomass and community composition by the phospholipid fatty acid (PLFA) method
The total microbial PLFAs are widely used as an index of total microbial biomass (Nikolaidou et al., 2021; Papatheodorou et al., 2021; Kapagianni et al., 2020). Extraction and analysis of phospholipid fatty acids from soil samples were performed within a week of post-sampling. Concisely, the procedure entails extraction of lipids; separation of phospholipids by column chromatography; methylation of esterified fatty acids in the phospholipid fraction; and chromatographic separation and identification of the main components on a Trace GC Ultra gas chromatograph (Thermo Finnigan, San Jose, CA) coupled with a Trace ISQ mass spectrometry (MS) detector, a split–splitless injector and an Xcalibur MS platform. Each fatty acid (nmol g−1) was quantified by one-point calibration against the GC response of the internal standard 19:0 methyl ester.
A total of 20 fatty acid methyl esters were identified and considered for further analysis, including the internal standard 19:0. The PLFA biomarkers were assigned to functional groups as follows: 15:0, i–15:0, a–15:0, i–16:0, 17:0 and i–17:0 (gram-positive bacteria) (McKinley et al., 2005); 16:1ω7c, 18:1ω9t and cyc17:0 (gram-negative bacteria) (Zhang et al., 2010); 10Me16:0, 10Me17:0 and 10Me18:0 (actinomycetes) (White et al., 1996); 18:2ω9,12 (fungi) (Rillig et al., 2006); 20:0 (microeukaryotes) (Smith et al., 1986). The PLFA biomarker 16:0 may derive from bacteria and fungi and 18:1ω9c from both gram-negative bacteria and fungi, while 11:0, 14:0 and 18:2ω6t are mainly of microbial origin (Rousidou et al., 2013; Monokrousos et al., 2021). The sum of all identified lipid quantities was used as an index of total microbial biomass, and the ratio isoanteiso was used as an index of environmental stress (Siliakus et al., 2017). We further estimated the ratios gramgram−, fungibacteria and saturatedunsaturated PLFAs.
2.5 Data analysis
To test the effects of BSC coverage regime and distance from the trunk of Erica spp. (0, 20, 40, 60, 80 and 100 cm) on water content, organic carbon, organic nitrogen, microbial biomasses and enzyme activity, we applied two-way ANOVA (analysis of variance) on data (n=60 for each parameter, 2 BSC coverage regimes × 6 distances from Erica spp. × 5 replicates). In cases where the data did not meet the assumptions of analysis of variance, a transformation was performed (PLFA values were logarithmically transformed, while the square transformation was applied on LAP and BG values). Analysis of variance was carried out using the Statistica 7.0 software.
To check for similarities in the composition of soil microbial communities collected at different sampling points, we applied one-way ANOSIM (analysis of similarities) on individual phospholipids' data sets with the Bray–Curtis index as a similarity index. The analyses were performed using PAST version 3.24 (Hammer et al., 2001).
A network analysis was applied to analyze the network of relationships between individual PLFA markers under BSC coverage regimes (presence and absence of crust) at three successive distances from the trunk of the Erica spp. shrubs. We applied network analysis on data collected from distances of 0–20, 40 and 60–100 cm. We divided the data collected along the line of 100 cm into three parts: those collected from a distance of 0–20 cm, where Erica had the greatest influence; at a distance of 40 cm, where the projection of the canopy of Erica was ended; and at a distance of >40 cm (60–100 cm). The last distance in crust-covered areas was covered by crust, while in uncrusted areas it was represented by bare soil. The nodes of the valued network represented PLFA biomarkers, while ties stood for the significant correlations between them (Stamou et al., 2019). The networks were analyzed with the Cytoscape and Ucinet packages and results visualized with an attribute circle layout.
Six networks were analyzed (2 BSC coverage regimes × 3 distance groups; 0–0.2, 0.40 and 0.60–1 m). In total, five network parameters were considered; three of them describe the cohesion of the network. These were density (the total of all correlation values divided by the number of possible ties), shortest path and clustering coefficient. Shortest path is the number of links contained in the shortest path that connects two nodes. Two nodes of a network could be connected by following different paths. They could be connected directly, the shortest path being 1; indirectly through another node, the shortest path being 2; indirectly through two other nodes, the shortest path being 3; etc. Thus, the mean shortest path of a network is the mean value of the lowest number of links that connect all pairs of nodes. The lower the shortest path, the more direct the connections between the nodes. The maximum value of compactness is 1, while smaller values indicate less compact networks. The clustering coefficient is the sum of the correlation coefficients between a node and its neighbors, and the weighted overall clustering coefficient is the weighted mean of the clustering coefficient of all nodes, each one weighted by its degree. Finally, the small-worldness index (SW) indicates whether a network exhibits small-world properties. This happens if the estimated length of shortest path (Lreal) is more or less equal to the shortest path (Lrand) estimated for an equivalent random network (Lreal≈Lrand), while the clustering coefficient of the network (Clreal) is much higher than that for the random counterpart (Clreal>>Clrand), i.e., if SW (Humphries and Gurney, 2008; Stamou et al., 2019). For testing departure from randomness the outputs of each experimental network were tested against those provided by 999 corresponding Erdős–Rényi random networks with the same number of nodes and density by using Student's t test for a single sample and N>30.
3.1 Physicochemical variables
Distance had no effect on soil water content. However, significant differences in water content were observed in relation to biocrust coverage (p<0.001); BSC soils had higher mean water content by 50 % compared to uncrusted soils. Organic carbon was not affected by the distance, coverage regime and their interaction. In contrast, organic N was affected significantly by the combination crust coverage × distance (Kruskal–Wallis ANOVA; chi-square: 24.96; p<0.01); significantly high soil N was recorded at distances of 0 and 20 cm in BSC soils, and significantly low soil N was recorded at a distance of 100 cm in soils without crust coverage.
3.2 Microbial biomass and community composition
Soil microbial biomass of bacteria, fungi, actinomycetes and microeukaryotes was not affected by the combination of crust coverage × distance. Biocrust coverage per se significantly affected the biomass of all microbial groups and their ratios, except the fungibacteria ratio (Table 1); higher microbial biomasses were recorded in uncrusted soils. In contrast, the ratios gramgram− bacteria and saturatedunsaturated PLFAs displayed higher values in crusted soils. Distance affected the total biomass, the biomass of actinomycetes and that of bacteria as well as the isoanteiso ratio. The lowest bacterial and actinomycete biomass was recorded at the base of Erica shrubs (at a distance of 0 cm) and the highest at a distance of 40 cm in both crusted and uncrusted soils. The isoanteiso ratio was low until the distance of 60 cm but increased at larger distances (Fig. 1).
Table 1Effect of the crust presence or absence (“Crust coverage”), distance (0, 20, 40, 60, 80 and 100 cm), and their interaction (“Coverage × distance”) on the biomass of various microbial groups. The asterisk indicates the level of significance (∗: p<0.05; : p<0.01; : p<0.001; and ns: not statistically significant). All microbial groups are expressed in nanomoles per gram.
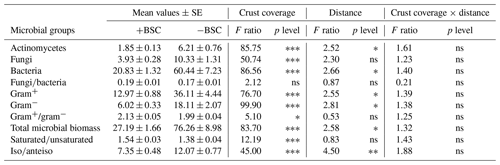
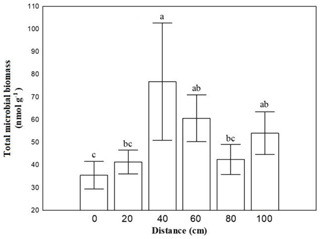
Figure 1Mean values (± SE) of soil microbial biomass at 0, 20, 40, 60, 80 and 100 cm distance, respectively, from the trunk of Erica spp. The letters a, b, c and d indicate the significant differences between distances revealed by a least squares distance (LSD) test.
ANOSIM examined similarities in the composition of the microbial communities sampled at different distances from the base of Erica spp. in crusted and uncrusted soils. The analysis revealed significant dissimilarities in communities' composition in both coverage regimes (Table 2). Each soil point has a distinct microbial community.
Table 2ANOSIM (p values) applied on individual PLFAs based on the Bray–Curtis index of similarity to detect dissimilarities in microbial community composition between different local communities (at different distances from the trunk of Erica spp. shrubs in crust-covered (+BSC) and uncrusted (−BSC) soils). For p values <0.05 dissimilarities are statistically significant.
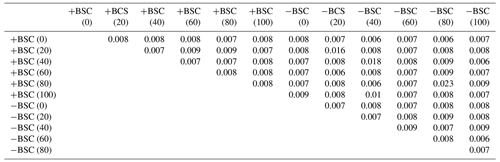
3.3 Enzyme activity
Similar to microbial abundances, no combined effect was detected for soil enzymes as well. Almost all soil enzymes responded to coverage status (Table 3). The majority of them presented higher values in areas covered with BSC, except LAP, which responded conversely. Distance affected only the activity of urease; lower values were recorded at distances larger than 60 cm (Fig. 2).
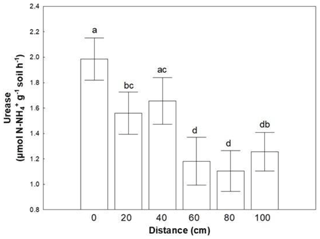
Figure 2Mean values (± SE) of urease activity at 0, 20, 40, 60, 80 and 100 cm distance, respectively, from the trunk of Erica spp. The letters a, b, c and d indicate the significant differences between distances revealed by an LSD test.
Table 3Mean values of enzyme activity (± SE) and effect of crust coverage (presence, +BSC, and absence,−BSC, of crust), distance (0, 20, 40, 60, 80 and 100 cm away from trunk of Erica spp.) and their interaction on soil activity (AP: acid phosphomonoesterase; BG: b-glucosidase; POD: peroxidase; PPO: polyphenol oxidase; NAG: N-acetylglucosaminidase; LAP: leucine aminopeptidase; and UR: urease). The asterisk ∗ indicates the level of significance (∗ p<0.05, p<0.01, p<0.001 and ns: not statistically significant).
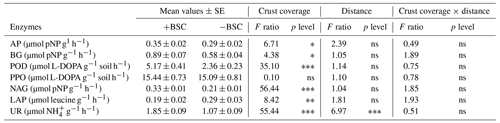
3.4 Microbial networks
The networks in soils covered only by Erica spp. (−BSC) showed a gradual increase in their topological features, with the exception of the shortest path as the distance from the trunk increased (Table 4). The network recorded at the distance until 20 cm was the least connected, exhibiting the lowest values in the estimates of density, compactness and weighted clustering coefficient and the highest value of the shortest path compared to the values recorded in networks of distances larger than 20 cm. The high value of the shortest path denotes mainly indirect connections between the nodes. Contrary to the networks recorded in uncrusted soils, in crusted ones (+BSC) the most connected network was that at a distance of 0–20 cm. This network presented the highest density and weighted clustering coefficient and the lowest average shortest path compared to networks recorded at distances larger than 20 cm. As concerns the small-worldness index, its highest value (5.11) was estimated for the network in crusted soil at 40 cm. Small values of the small-worldness index were recorded in almost all networks, while the network in uncrusted soil at distances larger than 60 cm did not show characteristics of small-worldness.
Table 4Values of certain global metrics estimated for the network of interactions between individual PLFAs under two crust coverage regimes at successive distances from the trunk to the periphery of Erica spp. shrubs. Comparisons of metrics with those produced by random networks with the same number of ties and nodes are also presented. In all cases the differences between real (underlined values) and random (Ran) networks were significant, with the exception of average distance and compactness estimated for uncrusted soils at a distance of 60–100 cm.
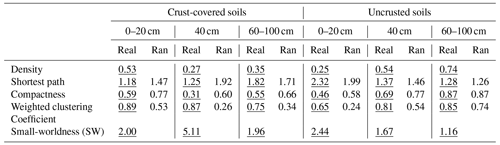
Biological crusts play an important role in plants' establishment and growth and vice versa. In this study, we examined the influence of the interplay between Cladonia rangiformis and Erica spp. and whether the spatial heterogeneity created by their interactions affected the biomass, the composition and the network of interactions among the members of the soil microbial communities. Soil water content was higher in areas covered by BSC than in those where biocrust was absent. By definition, BSC is a cohesive layer that can retain higher levels of moisture (Pointing and Belnap, 2012). Although it is frequently reported that BSCs resulted in significant stocks of soil C and N in arid and semiarid areas (Chamizo et al., 2012), this was not reported in this study. Gao et al. (2010) found that BSCs had no effect on these parameters at deep soil depths, suggesting that the effect of BSCs on soil carbon and nitrogen is restricted to the upper soil layer (up to 5 cm). This is probably the explanation for the lack of effect in our study since the samples were collected from the upper 10 cm of the soil.
The saturatedunsaturated ratio is indicative of the concentration of carbon or nutrients in the soil and increases as the concentration of nutrients decreases (Fierer et al., 2003). This ratio was higher in soils covered both by Erica and BSCs, denoting possible nutrient limitation in these soils and implying competition between C. rangiformis, Erica shrubs and soil microbes for nutrient uptake. It seems that, although biological crusts are known for their ability to fix atmospheric N, a fact that was in agreement with the higher organic N recorded at the base of the shrubs (0 cm distance) in crusted soils, this was not adequate to alleviate nutrient limitations. Further, the changes in saturatedunsaturated ratio could indicate changes in the composition of the microbial community. A positive relation between the gramgram− ratio and the saturatedunsatured ratio was mentioned by Konstantinou et al. (2019) and is based on the fact that most gram-positive bacteria contain saturated PLFAs (Cho and Salton, 1966). The changes in community composition were supported by changes in the gramgram− ratio, which was increased in crusted soils. This increase could be attributed to the decreased C availability or the exhaustion of labile substrates (Fanin et al., 2019). Given that the area is covered by the drought-tolerant evergreen sclerophyllous Erica spp., which exhibit slow rates of nutrient recycling (Mediavilla and Escudero, 2003), the low labile carbon in crusted soils could be attributed to consumption of labile C by the BSC microorganisms. The latter, in combination with the fact that through fungal metabolism lichens produce phenolic compounds which are considered recalcitrant, could explain the increase in the gramgram− ratio. Furthermore, other possible explanations for the increased gramgram− ratio could be due to the resistant (against exogenous factors) cell wall of gram+ bacteria (Rohde, 2019) that protects bacteria from the excretions of the lichen.
In crusted soils significantly lower values of the isoanteiso ratio were recorded, implying better abiotic conditions (temperature, humidity) compared to uncrusted ones since a high value of this ratio is generally used as an environmental stress indicator (Pettersson and Baath, 2003). Bacteria could decrease the content of anteiso fatty acids when growing under adverse conditions to decrease the viscosity of their cytoplasmic membranes. The high values of the ratio in uncrusted soils could be the result of the harsh temperature or moisture conditions prevailing in these soils. In fact, the water content in uncrusted soils was half of those in crusted ones.
Despite better abiotic conditions prevailing in crusted soils, microbial biomasses were almost 4 times lower compared to soils covered only with Erica shrubs. The latter contrasts literature data reporting a positive relation between the abundance of microorganisms and the presence of BSC (Guan et al., 2018) in arid and semiarid soils due to crusts' influence on the distribution of soil moisture. Therefore, the reduction in microbial biomasses in the present study may be explained as a negative by-product of Cladonia activity. It is thought that the allelochemicals' secondary metabolites of this organism containing aliphatic, aromatic and terpenoid halogenated components are involved in various chemical interactions, responsible for their antimicrobial properties (Kosanić et al., 2014). This idea is reinforced by a number of studies that show the negative effect of these lichens' substances on the biomass of soil microbes, tested both in the field and the laboratory (Pandey, 2017; Kalra et al., 2021).
Soil enzyme activity displayed an opposite pattern compared to that of microbial biomass, with higher values in crusted soils except for LAP and PPO. Besides, it is well documented that enzymes originate from a variety of sources such as living and dead microbes, plant roots, residues and soil animals (Sun et al., 2013). Thus, enzyme activity is not necessarily associated with microbial biomass. Ghiloufi et al. (2019) has reported that enzyme activity is high in soils covered by BSC because in most cases the presence of crusts is associated with high soil moisture, nutrients and organic matter content. In our study, by the inspection of the saturatedunsaturated ratio we identified nutrient limitation in soils covered by crust and Erica shrubs. Hence, soil microbial communities possibly produced enzymes for substrate degradation to increase nutrient availability for their nutritional needs according to the nutrient allocation theory (Allison and Vitousek, 2005). Also, if we consider the enzyme activity per unit of microbial biomass, this was much higher in the microbes of crusted soils, indicating more metabolically active microbial communities. The latter could be partially supported by the better abiotic conditions prevailing in these soils.
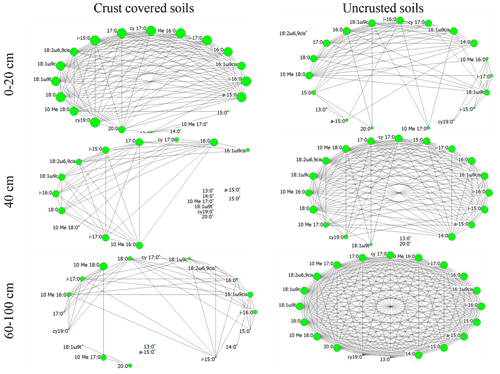
Figure 3Network of interactions among the members of the soil microbial community (various PLFA biomarkers) under two crust coverage regimes, along a straight line of 100 cm at successive distances from the trunk to the periphery of Erica spp. shrubs. The size of each node represents its eigenvector centrality; the larger the node the higher the eigenvector centrality and the higher the influence of the corresponding node.
It is interesting that spatial heterogeneity had a limited effect on microbial biomasses and enzyme activity. We observed a common pattern concerning the effect of distances on the microbial biomass, regardless of the presence of BSC. The microbial groups that were affected negatively by shrub presence were bacteria and actinomycetes, which displayed the lowest biomass at 0 cm distance from the shrubs and the highest at 40 cm. The fact that bacteria exhibited some kind of spatial heterogeneity is probably related to their limited ability to move and absorb nutrients. The active modes of their dispersal are limited to the scale of (connected) soil pores or aggregates (Yang and van Elsas, 2018). In contrast, the hyphal growth form of fungi likely conveys several advantages to these organisms, chief of which is the translocation of nutrients and resources from microsites where they are abundant to sites where they are limiting (Frey et al., 2003). As concerns the decrease in total microbial biomass at the shrub base, this could be attributed to the roots' increased density underneath the trunk, resulting in stronger competition for nutrients. Furthermore, phenolic compounds, which seem to have antimicrobial effects, are abundant in Ericaceae species (Malik, 1995). In some Ericaceae species such as E. andevalensis, a variety of phenols and flavonoids have been isolated and have proven to have allelopathic antimicrobial activity (Marquez-Garcia et al., 2009). Also, it has been found that this plant requires an acidic soil environment for its growth; however, this characteristic may provoke a quite inhospitable environment for the majority of soil microorganisms (Vazquez et al., 2020).
Beside quantitative measurements such as biomass and activity that displayed limited spatial heterogeneity, the composition of the local microbial communities was totally different from one point to another. At each soil point along a line of 100 cm from the trunk to the periphery of the shrubs, unique microbial communities were identified. This can be viewed as an effect of the fine-grained mosaic that characterizes the Mediterranean ecosystems (Stamou, 1998). The effect of spatial heterogeneity was mirrored at the microbial network topological features as well. The network in the area without cover (bare soils; Fig. 3f) was very well organized; the crust itself resulted in network fragmentation (Fig. 3c) probably due to released antimicrobial substances that acted selectively to the members of the microbial communities. Through negative effects on microbes, because of the acid soil environment and allelochemical substances contained in litter, the network in areas with only Erica seems to have collapsed (Fig. 3d). Remarkably, when both Erica and crust acted together (Fig. 3a), the microbial network was organized quite well as if the selective action of the crust on microbes were compensated by the selective action of Erica spp. or the opposite. Also, by comparing the networks in Fig. 3a and f we observed that the substances leached by Erica spp. and crust acted much more selectively for the microbial interactions than the adverse abiotic conditions prevailing in bare soil. The well-organized network in soils uncovered by Erica and crust soils with a high number of direct interconnections of relatively high intensity reflects a microbial community adapted to adverse abiotic conditions of the Mediterranean areas (Stamou et al., 1998). However, this was the only network that did not show characteristics of small-worldness. According to Peng et al. (2016) there is a trade-off between small-worldness and robustness. Thus, the organization of interactions between the members of the microbial communities in Mediterranean bare soils revealed them as the more robust. For these communities the risk of transition from stability to instability in the case of future disturbances such as trampling, fire or extreme watering is low. A similar response was reported by Papatheodorou et al. (2020) for the same Mediterranean area; the composition and the biomass of soil microbial communities in bare soils showed higher resistance to frequent-watering events than the communities in crust-covered soils.
We studied the effect of the spatial heterogeneity created by the crust C. rangiformis and the shrub Erica spp. on microbial biomass, community composition, network of interactions and enzyme activity in the soil. The quantitative measurements such as biomass and activity showed limited and no response to spatial heterogeneity, respectively; they changed mainly in relation to coverage regime. In contrast, the qualitative characteristics of the microbial communities such as their composition and networks' topology confirmed the idea of a fine-grained mosaic existing in the Mediterranean areas within distances of a few centimeters. Although the most well-organized and robust network was that in bare soils, it seems that Erica spp. and crust coverage acted in concert and conditioned the development of more connected networks compared to that produced by each agent alone. The interactions between soil microbial community, soil functionality, BSC and local vegetation are particularly complex. Hence, a multifaceted approach is recommended where qualitative and quantitative aspects are examined jointly.
The data are original and have been produced during this study. They are available at a public GitHub repository under the MIT license: https://doi.org/10.5281/zenodo.6411849 (papatheo1, 2022).
The supplement related to this article is available online at: https://doi.org/10.5194/we-22-21-2022-supplement.
TD and PDK conducted the soil analyses and wrote the original draft; NM analyzed the data; GPS and EMP conceived and designed the study; EMP wrote and edited the paper.
The contact author has declared that neither they nor their co-authors have any competing interests.
Publisher’s note: Copernicus Publications remains neutral with regard to jurisdictional claims in published maps and institutional affiliations.
This article is part of the special issue “Biological soil crusts”. It is not associated with a conference.
We would like to thank Eleni Zimvrakaki from the School of Agriculture, Aristotle University of Thessaloniki, for her contribution to PLFA analysis.
This paper was edited by Laura Concostrina-Zubiri and reviewed by two anonymous referees.
Allen, S. E.: Chemical analysis of ecological materials, Blackwell scientific publications, Oxford, UK, 1974.
Allison, S. D. and Vitousek, P. M.: Responses of extracellular enzymes to simple and complex nutrient inputs, Soil. Biol. Biochem., 37, 937–944, 2005.
Bainbridge, D.: A guide for desert and dryland restoration, Island Press, Washington DC, USA, 2012.
Bowker, M. A., Maestre, F. T., and Escolar, C.: Biological crusts as a model system for examining the biodiversity–ecosystem function relationship in soils, Soil Biol. Biochem., 42, 405–417, https://doi.org/10.1016/j.soilbio.2009.10.025, 2010.
Bu, C., Wu, S., Han, F., Yang, Y., and Meng, J.: The combined effects of moss-dominated biocrusts and vegetation on erosion and soil moisture and implications for disturbance on the Loess Plateau, China, PloS one, 10, e0127394, https://doi.org/10.1371/journal.pone.0127394, 2015.
Cania, B., Vestergaard, G., Kublik, S., Köhne, J. M., Fischer, T., Albert, A., Winkler, B., Schloter, M., and Schulz, S.: Biological Soil Crusts from Different Soil Substrates Harbor Distinct Bacterial Groups with the Potential to Produce Exopolysaccharides and Lipopolysaccharides, Microb. Ecol., 79, 326–341, https://doi.org/10.1007/s00248-019-01415-6, 2020.
Chamizo, S., Cantón, Y., Miralles, I., and Domingo, F.: Biological soil crust development affects physicochemical characteristics of soil surface in semiarid ecosystems, Soil Biol. Biochem., 49, 96–105, 2012.
Cho, K. Y. and Salton, M. R. J.: Fatty acid composition of bacterial membrane and wall lipids, BBA.-Lipid. Met., 116, 73–79, 1966.
Couradeau, E., Karaoz, U., Lim, H. C., Nunes Da Rocha, U., Northen, T., Brodie, E., and Garcia-Pichel, F.: Bacteria increase arid-land soil surface temperature through the production of sunscreens, Nat. Commun., 7, 1–7, https://doi.org/10.1038/ncomms10373, 2016.
Cullen, D. and Kersten, P. J.: Enzymology and molecular biology of lignin degradation, in: The Mycota III, edited by: Bramble, R. and Marzluf, G., Springer-Verlag Publishing, Germany, Berlin, 297–314, 1996.
Delgado-Baquerizo, M., Gallardo, A., Covelo, F., Prado-Comesaña, A., Ochoa, V., and Maestre, F. T.: Differences in thallus chemistry are related to species-specific effects of biocrust-forming lichens on soil nutrients and microbial communities, Funct. Ecol., 29, 1087–1098, https://doi.org/10.1111/1365-2435.12403, 2015.
Fanin, N., Kardol, P., Farrell, M., Nilsson, M.-C., Gundale, M. J., and Wardle, D. A.: The ratio of Gram-positive to Gram-negative bacterial PLFA markers as an indicator of carbon availability in organic soils, Soil Biol. Biochem., 128, 111–114, 2019.
Felse, P. A. and Panda, T.: Regulation and cloning of microbial chitinase genes, Appl. Microbiol. Biotechnol., 51, 141–151, https://doi.org/10.1007/s002530051374, 1999.
Fierer, N., Schimel, J. P., and Holden, P. A.: Variations in microbial community composition through two soil depth profiles, Soil Biol. Biochem., 35, 167–176, 2003.
Frey, S. D., Six, J., and Elliott, E. T.: Reciprocal transfer of carbon and nitrogen by decomposer fungi at the soil–litter interface, Soil Biol. Biochem., 35, 1001–1004, https://doi.org/10.1016/S0038-0717(03)00155-X, 2003.
Gao, S., Ye, X., Chu, Y., and Dong, M.: Effects of biological soil crusts on profile distribution of soil water, organic carbon and total nitrogen in Mu Us Sandland, China, J. Plant Ecol., 3, 279–284, 2010.
Ghiloufi, W., Seo, J., Kim, J., Chaieb, M., and Kang, H.: Effects of Biological Soil Crusts on Enzyme Activities and Microbial Community in Soils of an Arid Ecosystem, Microb. Ecol., 77, 201–216, https://doi.org/10.1007/s00248-018-1219-8, 2019.
Guan, P. T., Zhang, X. K., Yu, J., Cheng, Y. Y., Li, Q., Andriuzzi, S. W., and Liang, W. J.: Soil microbial food web channels associated with biological soil crusts in desertification restoration: The carbon flow from microbes to nematodes, Soil Biol. Biochem., 116, 82–90, https://doi.org/10.1016/j.soilbio.2017.10.003, 2018.
Hammer, Ø., Harper, D. A. T., and Ryan, P. D.: Past: paleontological statistics software package for education and data analysis, Palaeontol. Electronica, 4, 1–9, 2001.
Havrilla, C., Chaudhary, B., Ferrenberg, S., Antoninka, A., Belnap, J., Bowker, M., Eldridge, D. J., Faist, A. M., Huber-Sannwald, E., Leslie, A. D., Rodriguez-Caballero, E., Zhang, Y., and Barger, N. N.: Towards a predictive framework for biocrust mediation of plant performance: a meta-analysis, J. Ecol, 107, 2789–2807, 2019.
Humphries, M. D. and Gurney, K.: Network “small-world-ness”: a quantitative method for determining canonical network equivalence, PloS One, 3, e0002051, https://doi.org/10.1371/journal.pone.0002051, 2008.
Kalra, R., Conlan, X. A., and Goel, M.: Lichen allelopathy: a new hope for limiting chemical herbicide and pesticide use, Biocontrol Sci. Technol., 31, 772–796, https://doi.org/10.1080/09583157.2021.1901071, 2021.
Kapagianni, P. D., Topalis, I., Gwynn-Jones, D., Menkissoglu-Spiroudi, U., Stamou, G. P., and Papatheodorou, E. M.: Effects of plant invaders on rhizosphere microbial attributes depend on plant identity and growth stage, Soil Res., 59, 225–238, https://doi.org/10.1071/SR20138, 2020.
Kidron, G. J., Xiao, B., and Benenson, I.: Data variability or paradigm shift. Slow versus fast recovery of biological soil crusts-a review, Sci. Total Environ., 721, 137683, https://doi.org/10.1016/j.scitotenv.2020.137683, 2020.
Knight, T. R. and Dick, R. P.: Differentiating microbial and stabilized b-glucosidase activity relative to soil quality, Soil Biol. Biochem., 36, 2089–2096, https://doi.org/10.1016/j.soilbio.2004.06.007, 2004.
Konstantinou, S., Monokrousos, N., Kapagianni, P., Menkissoglu-Spiroudi, U., Gwynn-Jones, D., Stamou, G. P., and Papatheodorou, E. M.: Instantaneous response of microbial communities to stress in soils pretreated with Mentha spicata essential oil and/or inoculated with arbuscular mycorrhizal fungus, Ecol. Res., 34, 1–10, https://doi.org/10.1111/1440-1703.12030, 2019.
Kosanić, M., Ranković, B., Stanojković, T., Rančić, A., and Manojlović, N.: Cladonia lichens and their major metabolites as possible natural antioxidant, antimicrobial and anticancer agents, Food Sci. Technol., 59, 518–525, 2014.
Liu, Y., Yang, H., Li, X., and Xing, Z.: Effects of biological soil crusts on soil enzyme activities in revegetated areas of the Tengger Desert, China, Appl. Soil Ecol. 80, 6–14, https://doi.org/10.1016/j.apsoil.2014.03.015, 2014.
Maier, S., Tamm, A., Wu, D., Caesar, J., Grube, M., and Weber, B.: Photoautotrophic organisms control microbial abundance, diversity, and physiology in different types of biological soil crusts, ISME J., 12, 1032–1046, 2018.
Mallik, F. M.: Conversion of temperate forests into heaths: role of ecosystem disturbance and ericaceous plants, Environ. Manage., 19, 675-684, 1995.
Marquez-Garcia, B., Fernandez, M. A., and Cordoba, F.: Phenolics composition in Erica sp. differentially exposed to metal pollution in the Iberian Southwestern Pyritic Belt, Bioresour. Technol., 100, 446–451, 2009.
McKinley, V. L., Peacock, A. D, and White, D. C.: Microbial community PLFA and PHB responses to ecosystem restoration in tallgrass prairie soils, Soil Biol. Biochem., 37, 1946–1958, https://doi.org/10.1016/j.soilbio.2005.02.033, 2005.
Mediavilla, S. and Escudero, A.: Leaf life span differs from retention time of biomass and nutrients in the crowns of evergreen species, Funct. Ecol., 17, 541–548, 2003.
Menon, M., Yuan, Q., Jia, X., Dougill, A. J., Hoon, S. R., Thomas, A. D., and Williams, R. A.: Assessment of physical and hydrological properties of biological soil crusts using X-ray microtomography and modeling, J. Hydrol., 397, 47–54, 2011.
Monokrousos, N., Argyropoulou, M. D., Tzani, K., Menkissoglou-Spiroudi, U., Boutsis, G., D'Addabbo, T., and Ntalli, N.: The Effect of Botanicals with Nematicidal Activity on the Structural and Functional Characteristics of the Soil Nematode Community, Agriculture, 11, 326, https://doi.org/10.3390/agriculture11040326, 2021.
Ngosong, C, Buse, T., Ewald, M., Richter, A., and Glaser, K.: Influence of management intensity and environmental conditions on microbiota in biological soil crust and crust-free soil habitats of temperate forests, Soil Biol. Biochem., 144, 107761, https://doi.org/10.1016/j.soilbio.2020.107761, 2020.
Nikolaidou, C., Monokrousos, N., Kapagianni, P. D., Orfanoudakis, M., Dermitzoglou, T., and Papatheodorou, E. M.: The effect of Rhizophagus irregularis, Bacillus subtilis and water regime on the multifunctionality of the plant-soil system: The case of Lactuca sativa Agronomy, 11, 2183, https://doi.org/10.3390/agronomy11112183, 2021.
Pandey, A.: Lichens: a resource chest of herbal antimicrobial compounds, Int. J. Theoretic Appl. Sci., 9, 137–146, 2017.
papatheo1: papatheo1/Theophilos-data: Biocrust data, Zenodo [data set], https://doi.org/10.5281/zenodo.6411849, 2022.
Papatheodorou, E. M., Papapostolou, A., Monokro usos, N., Jones, D.-W., Scullion, J., and Stamou, G. P.: Crust cover and prior soil moisture status affect the response of soil microbial community and function to extreme rain events in an arid area, Eur. J. Soil Biol., 101, 103243, https://doi.org/10.1016/j.ejsobi.2020.103243, 2020.
Papatheodorou, E. M., Monokrousos, N., Angelina, E., and Stamou, G. P.: Robustness of rhizosphere microbial communities of L. sativa originated from soils of different legacy after inoculation with Plant Growth Promoting Rhizobacteria, Appl. Soil Ecol., 167, 104028, https://doi.org/10.1016/j.apsoil.2021.104028, 2021.
Peng, G., Tan, S. Y., Wu, J., and Holme, P.: Trade-offs between robustness and small-world effect in complex networks., Sci. Rep., 6, 37317, https://doi.org/10.1038/srep37317, 2016.
Pettersson, M. and Baath, E.: Temperature-dependent changes in the soil bacterial community in limed and unlimed soil, FEMS Microbiol. Ecol., 45, 13–21, https://doi.org/10.1016/S0168-6496(03)00106-5, 2003.
Pointing, S. B. and Belnap, J.: Microbial colonization and controls in dryland systems, Nat. Rev. Microbiol., 10, 551e562, https://doi.org/10.1038/nrmicro2831, 2012.
Rillig, M. C., Mummey, D. L., Ramsey, P. W., Klironomos, J. N., and Gannon, J. E.: Phylogeny of arbuscular mycorrhizal fungi predicts community composition of symbiosis-associated bacteria, FEMS Microbiol. Ecol., 57, 389–395, https://doi.org/10.1111/j.1574-6941.2006.00129.x, 2006.
Rodríguez-Caballero, E., Cantón, Y., Chamizo, S., Afana, A., and Solé-Benet, A.: Effects of biological soil crusts on surface roughness and implications for runoff and erosion, Geomorphology, 145–146, 81–89, 2012.
Rohde, M.: The gram-positive bacterial cell wall, Microbiol. Spectr., 7, 7.3.10, https://doi.org/10.1128/microbiolspec.GPP3-0044-2018 2019.
Rousidou, C., Papadopoulou, E. S., Kortsinidou, M., Giannakou, I. O., Singh, B. K., Menkissoglu-Spiroudi, U., and Karpouzas, D. G.: Bio-pesticides: harmful or harmless to ammonia oxidizing microorganisms? The case of a Paecilomyces lilacinus-basednematicides, Soil Biol. Biochem., 67, 98–105, https://doi.org/10.1016/j.soilbio.2013.08.014, 2013.
Siliakus, M. F., van der Oost, J., and Kengen, S. W. M.: Adaptations of archaeal and bacterial membranes to variations in temperature, pH and pressure, Extremophiles, 21, 651–670, 2017.
Sinsabaugh, R. L., Reynolds, H., and Long, T. M.: Rapid assay for amidohydrolase (urease) activity in environmental samples, Soil Biol. Biochem., 32, 2095–2097, https://doi.org/10.1016/S0038-0717(00)00102-4, 2000.
Smith, G. A., Nickels, J. S., Kerger, B. D., John, D., Davis, J. D., Collins, S. P., Wilson, J. T., McNabb, J. F., and White, D. C.: Quantitative characterization of microbial biomass and community structure in subsurface material: a prokaryotic consortium responsive to organic contamination, Can. J. Microbiol., 32, 104–111, https://doi.org/10.1139/m86-022, 1986.
Stamou, G. P.: Arthropods of Mediterranean-type ecosystems, Springer-Verlag, Berlin, Heidelberg, ISBN 9783642797521, 1998.
Stamou, G. P., Monokrousos, N., Gwynn-Jones, D., Whiteworth, D. E., and Papatheodorou, E. M.: A polyphasic approach for assessing ecosystem connectivity demonstrates that perturbation remodels network architecture in soil microcosms, Microb. Ecol., 78, 949–960, https://doi.org/10.1007/s00248-019-01367-x, 2019.
Steven, B., Gallegow-Graves, L. V., Yeager, C., Belnap, J., and Kuske, C. R.: Common and distinguishing features of the bacterial and fungal communities in biological soil crusts and shrub root zone soils, Soil Biol. Biochem., 69, 302–312, https://doi.org/10.1016/j.soilbio.2013.11.008, 2014.
Stursova, M., Crenshaw, C. L., and Sinsabaugh, R. L.: Microbial responses to long-term N deposition in a semiarid grassland, Microb. Ecol., 51, 90–98, https://doi.org/10.1007/s00248-005-5156-y, 2006.
Sun, X., Gao, C., and Guo, L. D.: Changes in soil microbial community and enzyme activity along an exotic plant Eupatorium adenophorum invasion in a Chinese secondary forest, Chin. Sci. Bull., 58, 4101–4108, https://doi.org/10.1007/s11434-013-5955-3, 2013.
Turner, B. L., McKelvie, I. D., and Haygarth, P. M.: Characterization of water extractable soil organic phosphorus by phosphatase hydrolysis, Soil Biol. Biochem., 34, 27–35, https://doi.org/10.1016/S0038-0717(01)00144-4, 2002.
Vazquez, E., Benito, M., Espejo, R., and Teutscherova, N.: Response of soil properties and microbial indicators to land use change in an acid soil under Mediterranean conditions, Catena, 189, e104486, https://doi.org/10.1016/j.catena.2020.104486, 2020.
Weber, B., Wu, D., Tamm, A., Ruckteschler, N., Rodriguez-Caballero, E., Steinkamp, J., Meusel, H., Elbert, W., Behrendt, T., Sorgel, M., Cheng, Y., Crutzen, P. J., Su, H., and Poschl, U.: Biological soil crusts accelerate the nitrogen cycle through large NO and HONO emissions in drylands, Proc. Natl. Acad. Sci., 112, 15384–15389, https://doi.org/10.1073/pnas.1515818112, 2015.
White, D., Stair, J., and Ringelberg, D.: Quantitative comparisons of in situ microbial biodiversity by signature biomarker analysis, J. Ind. Microbiol. Biotechnol., 17, 185–196, https://doi.org/10.1007/BF01574692, 1996.
Williams, A. J., Buck, B. J., and Beyene. M. A.: Biological soil crusts in the Mojave Desert, USA: micromorphology and pedogenesis, Soil Sci. Soc. Am. J., 76, 1685–1695, 2012.
Wu, Y., Rao, B., Wu, P., Liu, Y., Li, G., and Li, D.: Development of artificially induced biological soil crusts in fields and their effects on top soil, Plant Soil, 370, 115–124, https://doi.org/10.1007/s11104-013-1611-6, 2013.
Yang, P. and van Elsas, J. D.: Mechanisms and ecological implications of the movement of bacteria in soil, Appl. Soil Ecol., 129, 112–120, https://doi.org/10.1016/j.apsoil.2018.04.014, 2018.
Zhang, C., Xu, J., Liu, X., Dong, F., Kong, Z., Sheng, Y., and Zheng, Y.: Impact of imazethapyr on the microbial community structure in agricultural soils, Chemosphere, 81, 800–806, https://doi.org/10.1016/j.chemosphere.2010.06.079, 2010.
Zhang, Y., Aradottir, A. L., Serpe, M., Boeken, B.: Interactions of biological soil crusts with vascular plants, in: Biological soil crusts: an organizing principle in drylands, Springer, Cham, 2016.