the Creative Commons Attribution 4.0 License.
the Creative Commons Attribution 4.0 License.
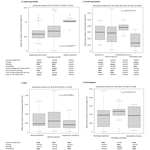
Little evidence for land-use filters on intraspecific trait variation in three arthropod groups
Katja Wehner
Matthias Brandt
Andrea Hilpert
Nadja K. Simons
Nico Blüthgen
Declines in species richness and abundance of insects over the last decades are often driven by anthropogenic land use and can have severe consequences for ecosystem functioning. Many studies investigated the effects of land-use intensification on the distribution of phenotypic traits across species at the community level, often with mixed results. However, biotic and abiotic environmental filters and potential selection act on individuals within each species, i.e., at the species' population level, and thus drive the extent of intraspecific phenotypic variation. Here, we compare the morphological trait variation within selected species of dung beetles, bees and grasshoppers and link this variation to land-use intensity in forests and grasslands. Selected traits included absolute body size measures and relative leg, wing or eye size, or shape and are often interpreted as “functional traits” in the context of specific ecological responses or effects. We predicted that trait variability among individuals of arthropod species is reduced in intensively used ecosystems (with pronounced environmental filtering) compared to low-intensity ones, particularly for arthropod species that were more abundant in intensively used sites (“land-use winners” compared to “losers”). In general, only few effects of land-use intensity on trait variation were found showing a decreasing variation with increasing land-use intensity in forests but an increasing variation in grasslands. Although many studies confirmed strong land-use impacts on species composition, diversity and trait distribution, including evidence from the same land-use gradients, we were not able to confirm consistent effects at the intraspecific level. However, the choice of which traits are included in analyses and the linkage between phenotypic variation and genetic variability can strongly influence the conclusions drawn on ecological processes. Therefore, we suggest extending the use of intraspecific trait variation on other, more specific response or effect traits and a broader range of species in future studies.
- Article
(1964 KB) - Full-text XML
-
Supplement
(3465 KB) - BibTeX
- EndNote
Insect decline and the rapid extinction of species, as well as consequences for species conservation, have become an important research topic (Thebault et al., 2014). Arthropod species extinction has been linked to both the change of climatic conditions (global warming) and the spatial extent and intensification of land use due to either direct mortality or indirect changes of biotopes (e.g., Loreau et al., 2001; Tilman et al., 2001; Potts et al., 2010; Barragán et al., 2011; Habel et al., 2019; Seibold et al., 2019). The loss of arthropod species and the decline of biodiversity have a major effect on retaining ecosystem functionality and resilience when environmental conditions change (e.g., Soliveres et al., 2016; Kühsel and Blüthgen, 2015; Loreau et al., 2001). This is especially true for arthropod species which are involved in many essential ecosystem services due to their high abundances and diversity (Samways, 1993; Kim, 1993). Some of these services maintain the stability of ecosystems, while others are beneficial for agricultural and forestry purposes, e.g., pollination, degradation of organic matter and bioturbation (Kim, 1993; Loreau et al., 2001; Losey and Vaughan, 2006).
In ecological studies on the effects of land use on insect communities, quantitative analyses of phenotypic traits have become widely used tools (Vandewalle et al., 2010; Rader et al., 2014; Simons et al., 2016; Mangels et al., 2017). Land-use intensification not only causes a general decline in diversity of arthropod communities but also influences the composition of so-called “functional traits” within communities (Loreau et al., 2001). These traits include specific physiological reaction norms, life-history traits, and often morphological structures that may be related to each species' functional performance or responses to their environment (deCastro-Arrazola et al., 2022). Species body size or body appendices are commonly used in correlation with land-use intensity (Simons et al., 2016; Birkhofer et al., 2017; Neff et al., 2019). Many studies found significant differences in functional traits among land-use types either within and/or across species; i.e., species with particular traits are replaced by other species with different traits (Moczek, 1998; Loreau et al., 2001; Barragán et al., 2011; Raine et al., 2018; Grass et al., 2021).
Most of the studies above focus on interspecific variation, i.e., on changes among arthropod species with changing environmental conditions or on differences between the trait distribution of arthropod communities in certain habitats. However, the importance of intraspecific variation – the trait distribution within a single species – is often neglected (Schindler et al., 2015; Bolnick et al., 2011; Violle et al., 2012; Mimura et al., 2016; Des Roches et al., 2018) even though selective pressures of biotic and abiotic environmental factors act on the individual level (Bolnick et al., 2011; de Bello et al., 2011; Violle et al., 2012; Grass et al., 2021; Chacón-Labella et al., 2022). In addition, trait changes through human activity (i.e., harvesting, pollution) can drive phenotypic plasticity and contemporary evolution, having effects at the population, community, and ecosystem level (Palkovacs et al., 2011, 2018; Grass et al., 2021). While environmental changes often harm poorly adapted populations, those with the lowest intraspecific variation (Mimura et al., 2016), high intraspecific phenotypic (and genetic) plasticity functions as a buffer and stabilizes ecosystem processes (Prieto et al., 2015). Furthermore, phenotypically heterogeneous species use a wider range of resources, which increases ecosystem productivity and nutrient cycling (Mimura et al., 2016). Hence, focusing on variation among individuals of the same species can help to identify mechanisms behind the effect of anthropogenic changes to ecosystem functions (if only certain traits show a change in variability). Additionally, potential threats to ecosystem resilience under changing conditions (if traits show lower variability with higher environmental change) can be visible.
In the present study, we examine functional morphological traits in response to land-use intensity in forests and grasslands of three arthropod groups with different ecological importance: dung beetles, bees, and grasshoppers. Dung beetles play an important role in mammalian dung removal. Especially tunneling genera such as Geotrupes, Anoplotrupes, and Onthophagus enhance soil quality due to dung decomposition, recycle nutrients, and reduce possible habitats for pest species due to their coprophagous feeding habit (Losey and Vaughan, 2006; Nichols et al., 2008; Frank et al., 2017). While measurements of eye and legs can be used to predict dung beetles' nesting behavior (Raine et al., 2018), their body size is frequently investigated in studies on the effects of land-use management (e.g., Barragán et al., 2011; Frank et al., 2017). Apart from morphometric measurements, numerous other types of traits for dung beetles have been defined (Buse et al., 2018; deCastro-Arrazola et al., 2022), and a recent review identified 136 trait–response and 77 trait–effect relationships across studies.
Pollinators such as wild bees have been widely studied and are known to suffer from habitat loss, habitat fragmentation, agrochemicals, pathogens, and alien species (e.g., Potts et al., 2010; Goulson et al., 2011; Lentini et al., 2012; Weiner et al., 2014; Kämper et al., 2017). The loss of pollination services has negative ecological and economic impacts for crops and wildflowers and affects the maintenance of wildflower diversity, ecosystem stability, and food security for human welfare (Potts et al., 2010; Gallai et al., 2009). Interspecific trait analyses of morphological (e.g., body or wing size) and feeding characters in pollinators indicate that environmental changes impact different kind of traits on different levels (Kämper et al., 2017; Kühsel, 2015; Gavini et al., 2019; Habel et al., 2019; Grass et al., 2021); i.e., body size and its variation increased with urbanization in generalist bees (Theodorou et al., 2020), potentially leading to reduced flower visitations (Gavini et al., 2019).
Grasshoppers are important herbivores in grasslands (Blumer and Diemer, 1996). Due to their feeding behavior they regulate plant communities (Zhang et al., 2011), influence nutrient cycling by enhancing decomposing plant biomass (Samways, 1993), and function as prey for other animals (Ingrisch and Köhler, 1998). Many species have been shown to be sensitive to environmental changes by land-use management such as fertilization, mowing and grazing (Chisté et al., 2016), and a within-site homogenization of trait variation with increasing land-use intensity can be observed (i.e., species were characterized by smaller body sizes and higher dispersal abilities; Birkhofer et al., 2015). Body size as morphological trait integrates ecological and physiological compromises in response to local environments (Parsons and Joern, 2014).
The present study focuses on forests and grasslands in Germany, two habitat types which have a long history of human use in central Europe. Hence, communities in those habitats are adapted to some level of anthropogenic activity. Nevertheless, many of those species react to changes and especially intensification of land-use intensity with changes in their abundance or frequency (Weiner et al., 2014; Chisté et al., 2016; Frank et al., 2018). Traits that affect the performance of individuals drive the variation of their fitness. However, differences in abundances define the community trait frequency distribution, thereby influencing ecological processes (Chacón-Labella et al., 2022).
We assume that land-use intensification changes intraspecific trait variation in dung beetles, bees, and grasshoppers in the same way as interspecific trait variation by selecting for specific traits or trait characteristics; hence intraspecific variation should be negatively affected by land-use intensity. However, since not all arthropod species react similarly to land-use intensity, we expect to see different patterns of intraspecific trait variation between species that occur mostly on sites with high land-use intensity (termed “winners”), species that were more abundant on sites with low land-use intensity (“losers”), and species that show no change in occurrence with changing land-use intensity (“neutral”). We predicted that intraspecific variability would be negatively affected by land-use intensity since more intensively used landscapes are typically more homogenous in terms of structure, environmental conditions, and resources which may be associated with more restricted phenotypic plasticity within a population. For losers we predicted lower levels of trait variability than for winners and a stronger decrease of this variability with increasing land-use intensity.
2.1 Sampling site
All insect taxa were collected in three different regions of Germany: the Swabian Alb (ALB), the Hainich-Dün (HAI), and the Schorfheide (SCH). In these three regions, plots in forests and grasslands have been set up for experiments and observations within the framework of the Biodiversity Exploratory Project (http://www.biodiversity-exploratories.de, last access: 4 January 2023; Fischer et al., 2010). The Swabian Alb is a low-mountain range in south-western Germany (460–860 m a.s.l.; 09∘10′49′′–09∘35′54′′ E/48∘20′28′′–48∘32′02′′ N). The Hainich-Dün is a hilly region located in central Germany (285–550 m a.s.l.; 10∘10′24′′–10∘46′45′′ E/50∘56′14′′–51∘22′43′′ N), and the Schorfheide-Chorin is a glacially formed landscape in north-eastern Germany (3–140 m a.s.l.; 13∘23′27′′–14∘08′53′′ E/52∘47′25′′–53∘13′26′′ N). The Schorfheide is characterized by the lowest annual precipitation (520–580 mm), with a mean annual temperature of 6–7 ∘C. It is followed by the Hainich (630–800 mm, 6.5–8 ∘C) and the Swabian Alb (800–930 mm, 8–8.5 ∘C).
The plots within each region (50 m × 50 m in grasslands, 100 m × 100 m in forests) cover a gradient of management practices and land-use intensity for the respective region. Forest plots include intensively managed spruce or pine plantation, mixed forests, and unmanaged beech stands. The forest management index (Formi) is composed of three subindices (Kahl and Bauhus, 2014): proportion of harvested trees (Iharv), proportion of non-native trees (Inonat), and the proportion of dead wood showing saw cuts (Idwcut). Iharv describes the proportion of harvested tree volume within a stand and is estimated by the presence of cut stumps and calculated as the ratio of harvested volume to the sum of standing, harvested, and dead wood volume (Kahl and Bauhus, 2014). Inonat is estimated as the proportion of harvested, living, and dead wood volume of non-natural tree species to the sum volume of all tree species. Idwcut represents the proportion of dead wood with saw cuts to the total amount of dead wood (Kahl and Bauhus, 2014). Formi, Iharv, Inonat, and Idwcut were obtained from the BExIS database for the year 2016 (Table S1 in the Supplement).
Grassland plots include intensively mown and fertilized plots, pastures of different grazing intensity, and species-rich grasslands; their land-use components are described in Blüthgen et al. (2012). The land-use index (LUI) for grassland sites is also composed of three subindices: fertilization intensity (F; kg nitrogen ha−1 yr−1), mowing frequency per year (M), and livestock grazing (G; livestock units days of grazing ha−1 yr−1). Data were obtained from the BExIS database and averaged for the sampling years (Table S1).
2.2 Sampling method
Specimens used for morphological trait measurement were obtained from a species collection at TU Darmstadt. They were formerly collected during the Biodiversity Exploratories for the study of Weiner et al. (2014; bees), Chisté et al. (2016; grasshoppers) and Frank et al. (2018; dung beetles).
Dung beetles were collected in forest and grassland sites across all three regions in spring and summer in 2014 and 2015 as part of a study on global dung webs (Frank et al., 2018). Individuals were captured using dung-baited pitfall trap using dung of cow, horse, sheep, red deer, wild boar, and fox. Six pitfall traps were placed in a transect along the site margin of the plots for 48 h. After capture, specimens were identified to species level and stored at −18 ∘C. For trait measurements, beetles were transferred into ethanol (70 %).
Bees were collected in 2008 and 2012 from grassland sites across all three regions (39 plots in the Swabian Alb, 39 in the Hainich, and 41 in the Schorfheide) by Weiner et al. (2014). Plots were surveyed over 6 h by walking along a transect of 200 m × 3 m along the edge of the plot; collected specimens were identified with the help of experts (Weiner et al., 2014).
Grasshoppers were collected in 2014 from grassland sites of the Swabian Alb and Hainich-Dün region using a biocenometer (1 m × 1 m × 0.6 m), made from an aluminum frame covered with gauze, which was quickly placed on an area, preventing insects from fleeing (Chisté et al., 2016). Samples were frozen at −18 ∘C; specimens were determined to species level and prepared with insect needles.
2.3 Species selection
For morphological trait measurement, 13 different species belonging to large and small dung beetles, bees, and grasshoppers were chosen from the originally sampled species collection (Table 1). The selection was based on several criteria: (1) the species occurred in as many plots as possible, at least 10 in total; (2) selected morphological traits were still measurable; and (3) the species' status in their response to certain land-use components (i.e., mowing, grazing, and fertilization in grasslands; harvesting intensity, non-native trees, and anthropogenic tree mortality in forests). For the latter, each species' land-use niche in relation to each land-use component was calculated using the abundance-weighted mean (AWM) of the respective land-use component across all plots. This species-specific AWM was compared to a null model assuming that species can occur on every site with the same likelihood. Species with a smaller or higher AWM than expected by the null model were declared losers or winners, respectively (see Chisté et al., 2016, for more detailed information). For the comparison of morphological trait variation, species being neutral to land-use components were also included (Table 1). This method to study species-level land-use responses and to distinguish winners and losers has been widely applied in the context of the Biodiversity Exploratories (e.g., Chisté et al., 2016, 2018; Busch et al., 2019; Mangels et al., 2017; Frank et al., 2017; Wehner et al., 2021a, b).
2.4 Intraspecific morphological traits
Within each taxonomic group a specific set of morphological traits was measured (Table 2). Traits were chosen which describe overall body size and dimensions (e.g., pronotum length, intersegmental width), mobility (e.g., femur length, forewing length) or interaction with the environment (e.g., eye surface). Due to the large morphological differences between taxa, morphological traits which are typically used in the literature were selected for each taxon and differ even between large and small dung beetles.
In large dung beetles, five traits were measured using a caliper. The hindfemur length was measured thrice on each side of the body. The overall length was calculated using the sum of elytra and pronotum length. In total, 1931 individuals of Anoplotrupes stercorosus, 285 individuals of Trypocopris vernalis, and 23 individuals of Typhaeus typhoeus from 151 plots were measured (Table S2).
In small dung beetles, seven morphological traits were measured on the left-hand side of the individual using a Keyence VHX-500 digital microscope with a VH-Z20R/W/T objective and a diffuser to reduce glare. The overall length was calculated using the sum of abdomen and pronotum length. In total, 39 individuals of Aphodius ater, 105 individuals of A. depressus, 82 individuals of Onthophagus fracticornis, and 26 individuals of O. similis from 74 plots were measured (Table S2).
In bees, nine traits were measured with a caliper on both sides of the individual if applicable. Wing and eye surface were calculated by multiplying wing length by wing width or eye length by eye width, respectively. In total, 97 individuals of Bombus lapidarius, 78 individuals of B. sylvarum, and 84 individuals of Lasioglossum calceatum from 73 plots were measured (Table S2).
In grasshoppers, four traits were measured using a digital caliper by Pollin Electronic with an accuracy of 0.02 mm for measurements below 100 mm. All traits were measured twice per individual and on both sides of the body if applicable. In total, 371 individuals of Chorthippus parallelus, 68 individuals of C. biguttulus, and 20 individuals of Stenobothrus lineatus from 61 plots were measured (Table S2).
For statistical analyses, mean values of repeated trait measurements and from the left- and right-hand side were calculated. Furthermore, relative values from each trait were standardized on different absolute morphological traits. For big and small dung beetles, we standardized the overall body length, for bees the intersegmental width, and for grasshoppers the length of the pronotum. For large dung beetles, the trait “shape” was formed by dividing the overall length by elytra width, and for small dung beetles shape was calculated from the quotient of overall length (sum of pronotum and abdomen length) and the overall width (pronotum width).
Further, relative femur width of small dung beetles was not scaled by the overall length, but on the leg length itself. This was calculated using the quotient from the hind respectively mid femur width and the summed femur and tibia length. Additionally, two more relative leg traits, not scaled on the overall length, were used: (i) the relative leg length between the hind and mid leg of the left-hand body side, which was the quotient from hind and mid femur and tibia length and (ii) the relative femur width between hind and mid leg, which represented the quotient from hind-femur and mid left-femur width.
2.5 Statistical analysis
All statistical analyses were performed using the software R version 3.6.0 (R Core Team, 2020) using the packages “nlme” (Pinheiro et al., 2021), “lme4” (Bates et al., 2015), and “lmerTest” (Kuznetsova et al., 2017).
Our analyses focused on the within-plot variation versus the among-plot variation in correlation with the land-use intensity of the respective plot. As response variable we used (1) the coefficient of variation (CV) of the body size trait per species per plot (see Fig. S1 for the correlation of CV per plot and numbers of individuals per plot) and compare the mean CV per species among plots. For all other morphological traits, trait values were standardized using the respective size traits per individual per plot. For those relative traits, we calculated the PC scores of the first axis using a principal component analysis (PCA; Fig. S2) and used (2) the PC-score standard deviation (SD) per species per plot to analyze community variability within plots. We further used the mean SD of PC scores per species to link intraspecific variation to the winner/loser/neutral status of the species. Combining multiple traits in a multivariate trait matrix is commonly suggested (e.g., Mouillot et al., 2021; Chacón-Labella et al., 2022). Since not all traits are equal in terms of their influence on functionality, it is a useful tool to integrate collections of traits into a few significant axes of phenotypic variation (Chacón-Labella et al., 2022).
As explanatory factors, land-use components such as the proportion of tree harvesting; the proportion of non-native trees; and the proportion of dead wood with saw cuts in forests and grazing, mowing, and fertilization in grasslands were used. Region and plot or region were implemented as a (nested) random factor. Since large dung beetles and grasshoppers have been sexed (male and female), the factor “sex” was also implemented as a random factor for these groups.
Table 3Effects of land-use components in forests and grasslands on the within-plot variation expressed as coefficient of variation of total body length in large and small dung beetles, bees, and grasshoppers.
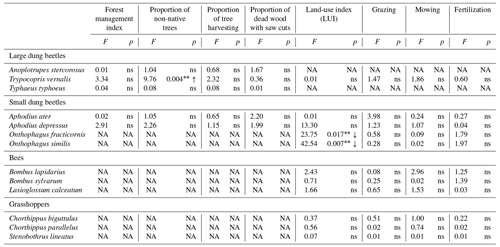
Abbreviations: ns – not significant (p > 0.05), NA – not available, = p ≤ 0.01, ↑ – increasing.
Before performing statistical analyses, data were tested for normal distribution and variance homogeneity using a Shapiro–Wilk test and a Levene test, respectively. If required, data were log 10 transformed to ensure normality and/or variance homogeneity. Statistical analyses were performed using linear mixed effect models (lmer) with the respective random factor(s). For species with < 50 individuals, i.e., Typhaeus typhoeus, Aphodius ater, Onthophagus similis, and Stenobothrus lineatus, we used a weight function in the linear models for weighting plots according to species abundance.
The intraspecific within-plot variation (CV) of body size ranged from 0 to 0.15 in large and small dung beetles, from 0 to 0.25 in grasshoppers, and was the highest in bees (< 1, Fig. 1). Among large dung beetles, the intraspecific within-plot size variation was significantly lower in Anoplotrupes stercorosus and Trypocopris vernalis than in Typhaeus typhoeus (Fig. 1a). In small dung beetles, bees, and grasshoppers, no differences of within-plot variation of body size among species was observed (Fig. 1b–d). The among-plot variation was generally lower than within-plot variation, ranging from 0.016 in Typhaeus typhoeus (large dung beetles) to 0.069 in Chorthippus biguttulus (grasshoppers).
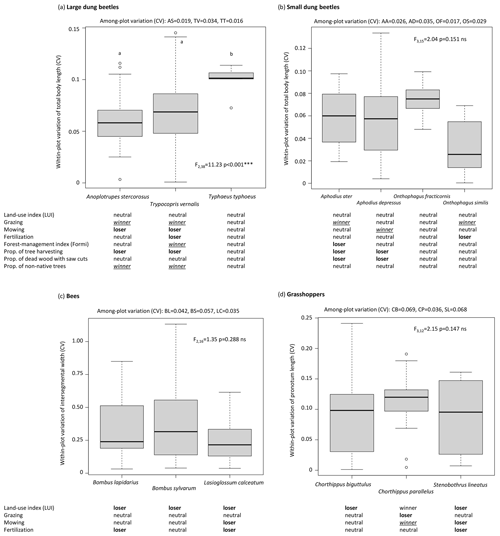
Figure 1Differences in within-plot variation of body-size traits in (a) large dung beetles, (b) small dung beetles, (c) bees, and (d) grasshoppers. Among-plot variation is shown above the plots, the species' status to the respective land-use component underneath. Note the different scales. Abbreviations: CV – coefficient of variation, ns – not significant (p > 0.05), ∗ = p ≤ 0.05, = p ≤ 0.001.
The impact of land-use intensity on within-plot body size variation within each species was generally weak (Table 3). In forests, within-plot variation of body size increased with an increasing proportion of non-native trees in T. vernalis (large dung beetles). Contrarily, it decreased with increasing land-use intensity in Onthophagus fracticornis and O. similis (small dung beetles) in grasslands. Variability of body size in bees and grasshoppers was not affected by any land-use component.
Absolute values of morphological traits were standardized as relative values to the respective body size before they were summarized as principal components (PCs) in analyses. In the three large dung beetle species, the relative length of the elytra mainly described the first axis, which explained 47.3 %, 72.8 %, and 58.4 % of the variation, respectively. In small dung beetle species, the first axis (explaining 58.5 %, 51.6 %, 60.9 %, and 58.6 % of the variation, respectively) was mainly described by relative traits of the hind leg (length and femur width; Fig. S2). Traits of the relative eye and wing surface described the first PC axis in bee species, explaining 52.3 %, 51.9 %, and 47.7 % of the variation, respectively. In grasshoppers, the first axis was mainly described by the relative forewing and pronotum length, explaining between 49.1 % and 61.9 % of the variation (Fig. S2).
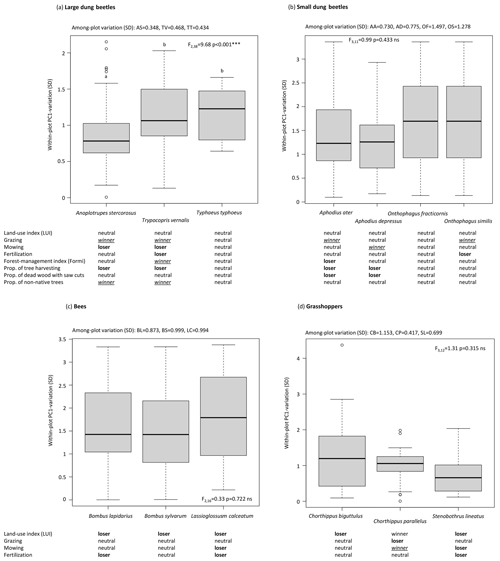
Figure 2Differences in within-plot variation of relative morphological traits by the principal component (PC1) scores in (a) large dung beetles, (b) small dung beetles, (c) bees, and (d) grasshoppers. Among-plot variation is shown above the plots, the species' status to the respective land-use component underneath. Abbreviations: SD – standard deviation, ns – not significant (p > 0.05), = p ≤ 0.001.
In large dung beetles, the intraspecific within-plot variation, expressed by the SD of PC scores, was significantly lower in A. stercorosus than in T. vernalis and T. typhoeus, which was also true for the among-plot variation (Fig. 2a). Species of small dung beetles, bees, and grasshoppers did not differ in their within-plot trait variation (Fig. 2b–d). Species of bees differed neither in their within-plot nor in their among-plot variation of relative trait values (Fig. 2c). Although not significant, C. biguttulus showed the highest within- and among-plot variability in grasshoppers (Fig. 2d).
Table 4Effects of land-use components in forests and grasslands on the within-plot variation of relative morphological traits expressed by the standard deviation of PC1 scores in large and small dung beetles, bees, and grasshoppers.
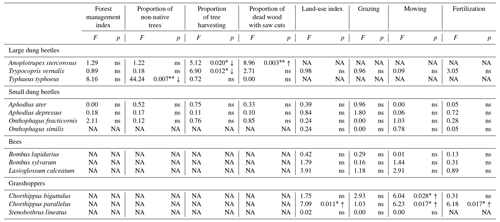
Abbreviations: ns – not significant (p > 0.05), NA – not available, ∗ = p ≤ 0.05, = p ≤ 0.01, ↑ – increasing, ↓ – decreasing.
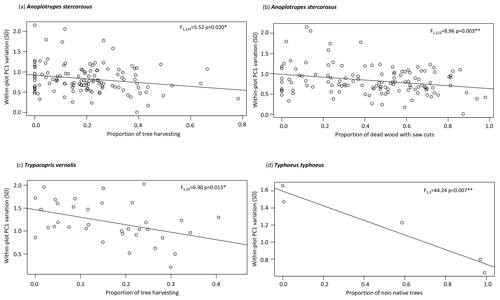
Figure 3Within-plot variation of relative morphological PC scores in (a–b) Anoplotrupes stercorosus, (c) Trypocopris vernalis, and (d) Typhaeus typhoeus in relation to the proportion of tree harvesting, dead wood with saw cuts, and non-native trees. Note the different scales. Abbreviations: ∗ = p ≤ 0.05.
The effect of land-use intensity on the intraspecific within-plot variation of relative morphological traits was generally weak and differed among habitats (Table 4). In forests, only species of large dung beetles have been significantly affected. Intraspecific trait variation decreased with increasing proportion of tree harvesting in A. stercorosus and T. vernalis (Fig. 3a, c), with increasing proportion of dead wood with saw cuts in A. stercorosus (Fig. 3b) and with increasing proportion of non-native trees in Typhaeus typhoeus (Fig. 3c, Table 4). In grasslands, effects were only present in grasshoppers (Table 4); the increase in intensity of land-use factors (except grazing) always caused an increasing within-plot variability of relative traits (Fig. 4a–d, Table 4).
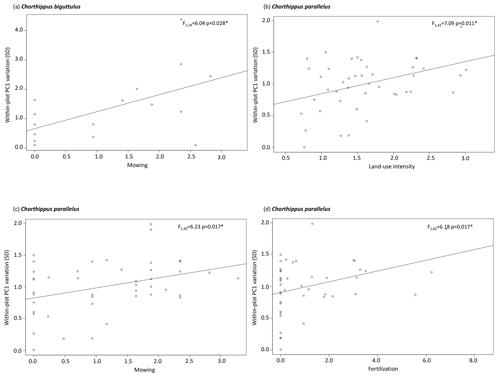
Figure 4Within-plot variation of relative morphological PC scores in (a) Chorthippus biguttulus and (b–d) Chorthippus parallelus in relation to mowing, fertilization, and land-use index (LUI). Note the different scales. Abbreviations: ∗ = p ≤ 0.05.
Contrary to our prediction, the degree of within-plot variation of relative morphological traits was independent of the winner or loser status for all land-use components except the proportion of non-native trees in forests: here, winner species showed a significantly lower variability than those classified as neutral (Fig. 5).
We compared the extent of intraspecific trait variation and the potential impact of increasing land-use intensity among arthropod groups representing different ecological functions. Our results confirmed species-specific differences in morphometric variation in body size and relative size of traits. However, land-use intensity provided little or no explanation for intraspecific trait variation in body size related traits for the arthropod taxa selected in our study. Furthermore, the direction of effects differed in forests and grassland habitats.
However, examples from literature show effects of environmental parameters (e.g., land use, predation pressure, and latitude) on size traits in various species. In generalized pollinators, body size is a key trait linked to metabolism and dispersal. At the community level, body size and its variation increased with urbanization (Theodorou et al., 2021), but overall size diversity decreased with landscape simplification (Grass et al., 2021). In dung beetles, larger bodied species were more vulnerable to effects of habitat disturbance (Raine et al., 2018) and functional diversity decreased with increasing land use intensity (Barragán et al., 2011).
The variation of combined relative morphological traits was also only weakly affected by land use and again the direction of effects differed among habitats; i.e., variation decreased in forests but increased in grasslands. Almost all effects were independent of the winner or loser status of the species; only on plots with a high proportion of non-native trees were winner species less variable than those that were neutral. Therefore, we could not finally confirm our prediction that the intraspecific variation is negatively affected by increasing land-use intensity due to a narrow adaptation of winner species to harsh environmental conditions.
Anthropogenic disturbances are assumed to cause evolutionary changes in phenotypic plasticity (Loreau et al., 2001; Palkovacs et al., 2011; Violle et al., 2012; Mimura et al., 2016; Crispo et al., 2010). The direction of these changes, however, depends on the interaction between taxon and trait, but an increase in plasticity following anthropogenic disturbances seems more common (Loreau et al., 2001; Crispo et al., 2010). However, in invertebrates plasticity in life-history traits increased while those for morphological traits decreased (Crispo et al., 2010). Additionally, phenotypic variation arises not necessarily from environmental filters, but from genotypic differences, or is indirectly affected by ecological factors such as a nutritionally imbalanced diet (Brückner et al., 2018).
We expected trait variation to decrease following constricting selection since high land-use intensity acts as a selective filter by homogenizing resources (Chistè et al., 2018). Thereby, the focus on intraspecific instead of interspecific variation aimed to include natural phenotypical trait variation within populations of a species that would otherwise be neglected (Albert et al., 2010; Bolnick et al., 2011). Intraspecific variation is important since ecological interaction depends on species' traits and its variation alters the interaction strength and can simultaneously protect populations from extreme temporal density fluctuations (Bolnick et al., 2011). However, the choice of which traits are included in analyses can drastically change the conclusions of ecological processes (Wong and Carmona, 2020; Chacón-Labella et al., 2022; Keller et al., 2022). Furthermore, management practices of long-term experiments on biodiversity–ecosystem functioning may influence the mechanistic linkage between environment and traits since community-weighted values of a given responsive trait shift with changes of relative abundances of the species and management practices do not allow community assembly processes to operate (Lepš et al., 2011; Chacón-Labella et al., 2022). Generally, shifts in trait distributions at the community levels, typically depicted by community-weighted means, may generally be more dynamic and responsive to environmental gradients since they mirror a shift in relative abundances of mobile animal species rather than changes in morphological traits themselves. Instead intraspecific trait variation is likely to be more conservative and less dynamic in response to small-scale environmental gradients reflected by land-use intensity.
Overall, we were not able to confirm that environmental filters, represented by anthropogenic land use for which strong effects on communities are known, had a similarly strong impact on an intraspecific phenotypic variation. However, we did not link phenotypic variation to genetic variability, nor to specific processes during juvenile development. Furthermore, some traits may evolve more rapidly than others, and also heritability of plasticity differs (Crispo et al., 2010). We thus suggest to expand the focus of intraspecific trait variation on different traits and a broader range of species in future studies.
The data used in this study can be obtained from sources cited in Table S1 in the Supplement and from the corresponding author upon request.
The supplement related to this article is available online at: https://doi.org/10.5194/we-23-35-2023-supplement.
NB conceived the study; MB and AH collected the data; KW, MB, and NKS analyzed the data; MB and KW wrote the original manuscript; all authors contributed substantially to the final manuscript.
The contact author has declared that none of the authors has any competing interests.
Publisher’s note: Copernicus Publications remains neutral with regard to jurisdictional claims in published maps and institutional affiliations.
We thank the managers of the three Exploratories (Miriam Teuscher, Anna Franke, Julia Bass, Max Müller, and Franka Mariam) and all former managers for their work in maintaining the plot and project infrastructure; Victoria Grießmeier for giving support through the central office; Andreas Ostrowski for managing the central database; and Markus Fischer, Eduard Linsenmair, Dominik Hessenmöller, Daniel Prati, Ingo Schöning, François Buscot, Ernst-Detlef Schulze, and the late Elisabeth Kalko for their role in setting up the Biodiversity Exploratories project. We thank the administration of the Hainich National Park, the UNESCO Biosphere Reserve Swabian Alb, and the UNESCO Biosphere Reserve Schorfheide-Chorin as well as all land owners for the collaboration.
Fieldwork permits were issued by the responsible state environmental offices of Baden-Württemberg, Thuringia, and Brandenburg. We thank a number of student helpers for contributing to fieldwork, Kevin Frank, Melanie Chisté and, Christiane Weiner for providing specimens, and Nadine Langner-Bürgin and Fabian Winter for trait measuring. Finally, we thank Michael Staab, Finn Rehling, and one anonymous reviewer for valuable comments on the manuscript.
The work has been partly funded by the DFG Priority Program 1374 “Biodiversity- Exploratories” (DFG-WE3081/21-4).
This paper was edited by Roland Brandl and reviewed by Finn Rehling and one anonymous referee.
Albert, C. H., Thuiller, W., Yoccoz, N. G., Douzet, R., Aubert, S., and Laorel, S.: A multi-trait approach reveals the structure and the relative importance of intra- vs. interspecific variability in plant traits, Funct. Ecol., 24, 1192–1201, 2010.
Barragán, F., Moreno, C. E., Escobar, F., Halffter, G., and Navarrete, D.: Negative impacts of human land use on dung beetle functional diversity, PlosOne, 6, e17976, https://doi.org/10.1371/journal.pone.0017976, 2011.
Bates, D., Mächler, M., Bolker, B., and Walker, S.: Fitting Linear Mixed-Effects Models Using lme4, J. Stat. Software, 67, 1–48, 2015.
Birkhofer, K., Smith, H. G., Weisser, W. W., Wolters, V., and Gossner, M. M.: Land-use effects on the functional distinctness of arthropod communities, Ecography, 38, 889–900, 2015.
Birkhofer, K., Gossner, M. M., Diekötter, T., Drees, C., Ferlian, O., Maraun, M., Scheu, S., Weisser, W. W., Wolters, V., Wurst, S., Zaitsev, A. S., and Smith, H. G.: Land-use type and intensity differentially filter traits in above-and below-ground arthropod communities, J. Anim. Ecol., 86, 511–520, 2017.
Blumer, P. and Diemer, M.: The occurrence and consequences of grasshopper herbivory in an alpine grassland, Swiss Central Alps, Arctic Alpine Res., 28, 435–440, 1996.
Blüthgen, N., Dormann, C. F., Prati, D., Klaus, V. H., Kleinebecker, T., Hölzel, N., Alt, F., Boch, S., Gockel, S., Hemp, A., Müller, J., Nieschulze, J., Renner, S. C., Schöning, I., Schumacher, U., Socher, S. A., Wells, K., Birkhofer, K., Buscot, F., Oelmann, Y., Rothenwöhrer, C., Scherber, C., Tscharntke, T., Weiner, C. N., Fischer, M., Kalko, E. K. V., Linsenmair, K. E., Schulze, E.-D., and Weisser, W. W.: A quantitative index of land-use intensity in grasslands: Integrating mowing, grazing and fertilization, Basic Appl. Ecol., 13, 207–220, 2012.
Bolnick, D. I., Amarasekare, P., Araújo, M., Bürger, R., Levine, J. M., Novak, M., Rudolf, V. H. W, Schreiber, S. J., Urban, M. C., and Vasseur, D. A.: Why intraspecific trait variation matters in community ecology, Trends Ecol. Evol., 4, 183–192, 2011.
Brückner, A., Schuster, R., Wehner, K., and Heethoff, M.: Nutritional quality modulates intraspecific trait variability, Front. Zool., 15, 50, https://doi.org/10.1186/s12983-018-0297-2, 2018.
Busch, V., Klaus, V. H., Schäfer, D., Prati, D., Boch, S., Müller, J., Chisté, M., Mody, K., Blüthgen, N., Fischer, M., Hölzel, N., and Kleinebecker, T.: Will I stay or will I go? Plant species-specific response and tolerance to high land-use intensity in temperate grassland ecosystems, J. Veg. Sci., 30, 674–686, 2019.
Buse, J., Šlachta, M., Sladecke, F. X. J., and Carpaneto, G. M.: Summary of the morphological and ecological traits of Central European dung beetles, Entomol. Sci., 21, 315–323, 2018.
Chacón-Labella, J., Hinojo-Hinojo, C., Bohner, T., Castorena, M., Violle, C., Vandvik, V., and Enquist, B. J.: How to improve scaling from traits to ecosystem processes, Trends Ecol. Evol., 3096, 1–10, 2022.
Chisté, M. N., Mody, K., Gossner, M. M., Simons, N. K., Kohler, G., Weisser, W. W., and Blüthgen, N.: Losers, winners, and opportunists: How grassland land-use intensity affects orthopteran communities, Ecosphere, 7, e01545, https://doi.org/10.1002/ecs2.1545, 2016.
Chisté, M. N., Mody, K., Knuz, G., Gunczy, J., and Blüthgen, N.: Intensive land use drives small-scale homogenization of plant and leafhopper communities and promotes generalists, Oecologia, 186, 529–540, 2018.
Crispo, E., DiBattista, J. D., Correa, C., Thibert-Plante, X., McKellar, A. E., Schwartz, A. K., Berner, D., De León, L. F., and Hendry, A. P.: The evolution of phenotypic plasticity in response to anthropogenic disturbance, Evol. Ecol. Res., 12, 47–66, 2010.
de Bello, F., Lavroel, S., Albert, C. H., Thuiller, W., Grigulis, K., Dolezal, J., Janeček, S., and Lepš, J.: Quantifying the relevance of intraspecific trait variability for functional diversity, Methods Ecol. Evol., 2, 163–174, 2011.
deCastro-Arrazola, I., Andrew, N. R., Berg, M. P., Curtsdotter, A., Lumaret, J.-P., Menéndez, R., Moretti, M., Nervo, B., Nichols, E. S., Sánchez-Piñero, F., Santos, A. M. C., Sheldon, K. S., Slade, E. M., and Hortal, J.: A trait-based framework for dung beetle functional ecology, J. Animal Ecol., 92, 44–65, https://doi.org/10.1111/1365-2656.13829, 2022.
Des Roches, S., Post, D. M., Turley, N. E., Bailey, J. K., Hendry, A. P., Kinnison, M. T., Schweitzer, J. A., and Palkovacs, E. P.: The ecological importance of intraspecific variation, Nature Ecol. Evol., 2, 57–64, 2018.
Fischer, M., Bossdorf, O., Gockel, S., Hansel, F., Hemp, A., Hessenmoller, D., Korte, G., Nieschulze, J., Pfeiffer, S., Prati, D., Renner, S., Schöning, I., Schumacher, U., Wells, K., Buscot, F., Kalko, E. K. V., Linsenmair, E., Schulze, E.-D., and Weisser, W. W.: Implementing large-scale and long-term functional biodiversity research: The Biodiversity Exploratories, Basic Appl. Ecol., 11, 473–485, 2010.
Frank, K., Hulsmann, M., Assmann, T., Schmitt, T., and Blüthgen, N.: Land use affects dung beetle communities and their ecosystem service in forests and grasslands, Agric. Ecosyst. Environ., 243, 114–122, 2017.
Frank, K., Krell, F. T., Slade, E. M., Raine, E. H., Chiew, L. Y., Schmitt, T., Vairappan C. S., Walter, P., and Blüthgen, N.: Global dung webs: high trophic generalism of dung beetles along the latitudinal diversity gradient, Ecol. Lett., 21, 1229–1236, 2018.
Gallai, N., Salles, J.-M., Settele, J., and Vaissière, B. E.: Economic valuation of the vulnerability of world agriculture confronted with pollinator decline, Ecol. Econom., 68, 810–821, 2009.
Gavini, S. S., Quintero, C., and Tadey, M.: Intraspecific variation in body size of bumblebee workers influences anti-predator behavior, J. Anim. Ecol., 89, 658–669, 2019.
Goulson, D., Rayner, P., Dawson, B., and Darvill, B.: Translating research into action; bumblebee conservation as a case study, J. Appl. Ecol., 48, 3–8, 2011.
Grass, I., Albrecht, J., Farwig, N., and Jauker, F.: Plant traits and landscape simplification drive intraspecific trait diversity of Bombus terrestris in wildflower plantings, Basic Appl. Ecol., 57, 91–101, 2021.
Habel, J. C., Ulrich, W., Biburger, N., Seibold, S., and Schmitt, T.: Agricultural intensification drives butterfly decline, Insect Conserv. Div., 12, 289–295, https://doi.org/10.1111/icad.12343, 2019.
Ingrisch, S. and Köhler, G.: Die Heuschrecken Mitteleuropas, Westarp Wissenschaften, Magdeburg, Germany, ISBN 3894324619, 1998.
Kahl, T. and Bauhus, J.: An index of forest management intensity based on assessment of harvested tree volume, tree species composition and dead wood origin, Nature Conserv., 7, 15–27, 2014.
Kämper, W., Weiner, C., Kühsel, S., Storm, C., Eltz, T., and Blüthgen, N.: Evaluating the effects of floral resource specialisation and nitrogen regualtion on the vulnerabilty of social bees in agricultural landscapes, Apidologie, 48, 371–383, 2017.
Keller, A., Ankenbrand, M. J., Bruelheide, H., Dekeyzer, S., Enquist, B. J., Bagher Erfanian, M., Falster, D. S., Gallagher, R. V., Hammnock, J., Kattge, J., Leonhardt, S. D., Madin, J. S., Maitner, B., Neyret, M., Onstein, R. E., Pearse, W. D., Poelen, J. H., Slaguero-Gomez, R., Schneider, F. D., Tóth, A. B., and Penone, C.: Ten (mostly) simple rules to future-proof trait data in ecological and evolutionary sciences, Methods Ecol. Evol., 14, 1–15, 2022.
Kim, K. C.: Biodiversity, conservation and inventory – Why insects matter, Biodiv. Conserv., 2, 191–214, 1993.
Kühsel, S.: Pollinator trait diversity: functional implications at different land-use intensities and environmental conditions, Disseration at the TU Darmstadt, http://tuprints.ulb.tu-darmstadt.de, last access: 31 December 2015.
Kühsel, S. and Blüthgen, N.: High diversity stabilizes the thermal resilience of pollinator communities in intensively managed grasslands, Nat. Commun., 6, 7989, https://doi.org/10.1038/ncomms8989, 2015.
Kuznetsova, A., Brockhoff, P. B., and Christensen, R. H. B.: lmerTest Package: Tests in Linear Mixed Effects Models, J. Stat. Softw., 82, 1–26, https://doi.org/10.18637/jss.v082.i13, 2017.
Lentini, P. E., Martin, T. G., Gibbons, P., Fischer, J., and Cunningham, S. A.: Supporting wild pollinators in a temperate agricultural landscape: Maintaining mosaics of natural features and production, Biol. Conserv., 149, 84–92, 2012.
Lepš, J., de Bello, F., Šmilauer, P., and Doležal, J.: Community trait response to environment: disentangling species turnover vs intraspecific trait variability effects, Ecography, 34, 856–863, 2011.
Loreau, M., Naeem, S., Inchausti, P., Bengtsson, J., Grime, J. P., Hector, A., Hooper, D. U., Huston, M. A., Raffaelli, D., Schmid, B., Tilman, D., and Wardle, D. A.: Biodiversity and ecosystem functioning: current knowledge and future challenges, Science, 294, 804–808, 2001.
Losey, J. E. and Vaughan, M.: The economic value of ecological services provided by insects, Bioscience, 56, 311–323, 2006.
Mangels, J., Fiedler, K., Schneider, F. D., and Blüthgen, N.: Diversity and trait composition of moths respond to land-use intensification in grasslands: generalists replace specialists, Biodiv. Conserv., 26, 3385–3405, 2017.
Mimura, M., Yahara, T., Faitha, D. P., Vázquez-Domínguez, E., Colautti, R. I., Araki, H., Javadi, F., Núñez-Farfán, J., Mori, A. S., Zhou, S., Hollingsworth, P. M., Neaves, L. E., Fukano, Y., Smith, G. F., Sato, Y.-I., Tachida, H., and Hendry, A. P.: Understanding and monitoring the consequences of human impact on intraspecific variation, Evol. Appl., 10, 121–139, 2016.
Moczek, A. P.: Horn polyphenism in the beetle Onthophagus taurus: larval diet quality and plasticity in parental investment determine adult body size and male horn morphology, Behav. Ecol., 9, 636–641, 1998.
Mouillot, D., Loiseau, N., Grenié, M., Algar, A. C., Allegra, M., Cadotte, M. W., Casajus, N., Denelle, P., Guéguen, M., Maire, A., Maitner, B., McGill, B. J., McLean, M., Mouquet, N., Munoz, F., Thuiller, W., Villéger, S., Violle, C., and Auber, A.: The dimensionality and structure of species trait spaces, Ecol. Lett., 24, 1988–2009, 2021.
Neff, F., Blüthgen, N., Chisté, M. N., Simons, N. K., Steckel, J., Weisser, W. W., Westphal, C., Pellissier, L., and Gossner, M. M.: Cross-scale effects of land use on the functional composition of herbivorous insect communities, Landscape Ecol., 34, 2001–2015, 2019.
Nichols, E., Spector, S., Louzada, J., Larsen, T., Amequita, S., Favila, M. E., and Network, S. R.: Ecological functions and ecosystem services provided by Scarabaeinae dung beetles, Biol. Conserv., 141, 1461–1474, 2008.
Palkovacs, E. P., Kinnison, M. T., Correa, C., Daltons, C. M., and Hendry, A. P.: Fates beyond traits: ecological consequences of human-introduced trait change, Evol. Appl., 5, 183–191, 2011.
Palkovacs, E. P., Moritsch, M. M., Contolini, G. M., and Pelletier, F.: Ecology of harvest-driven trait changes and implications for ecosystem management, Front. Ecol. Environ., 16, 20–18, 2018.
Parsons, S. M. A. and Joern, A.: Life history traits associated with body size covary along a latitudinal gradient in a generalist grasshopper, Oecologia, 174, 379–391, https://doi.org/10.1007/s00442-013-2785-6, 2014.
Pinheiro, J., Bates, D., DebRoy, S., Sarkar, D., and R Core Team: nlme: Linear and Nonlinear Mixed Effects Models, R package version 3.1-152, http://CRAN.R-project.org/package=nlme, last access: 17 April 2021.
Potts, S. G., Biesmeijer, J. C., Kremen, C., Neumann, P., Schweiger, O., and Kunin, W. E.: Global pollinator declines: trends, impacts and drivers, Trends Ecol. Evol., 25, 345–353, 2010.
Prieto, I., Violle, C., Barre, P., Durand, J., Ghesquiere, M., and Litrico, I.: Complementary effects of species and genetic diversity on productivity and stability of sown grasslands, Nat. Plants, 1, 15033, https://doi.org/10.1038/nplants.2015.33, 2015.
Rader, R., Bartomeus, I., Tylianakis, J. M., and Laliberté, E.: The winners and losers of land use intensification: Pollinator community disassembly is non-random and alters functional diversity, Div. Dist., 20, 908–917, 2014.
Raine, E. H., Gray, C. L., Mann, D. J., and Slade, E. M.: Tropical dung beetle morphological traits predict functional traits and show intraspecific differences across land uses, Ecol. Evol., 8, 8686–8696, 2018.
R Core Team: R: A language and environment for statistical computing, R Foundation for Statistical Computing, Vienna, Austria, https://www.R-project.org/, last access: 19 October 2020.
Samways, M. J.: Insects in biodiversity conservation – Some perspectives and directives, Biodiv. Conserv., 2, 258–282, 1993.
Schindler, D. E., Armstrong, J. B., and Reed, T. E.: The portfolio concept in ecology and evolution, Front. Ecol. Environ., 13, 257–263, 2015.
Seibold, S., Gossner, M. M., Simons, N. K., Blüthgen, N., Müller, J., Ambarli, D., Ammer, C., Bauhus, J., Fischer, M., Habel, J. C., Linsenmair, K. E., Nauss, T., Penone, C., Prati, D., Schall, P., Schulze, E.-D., Vogt, J., Wöllauer, S., and Weisser, W. W.: Arthropod decline in grasslands and forests is associated with landscape-level drivers, Nature, 574, 671–674, 2019.
Simons, N. K., Weisser, W. W., and Gossner, M. M.: Multi-taxa approach shows consistent shifts in arthropod functional traits along grassland land-use intensity gradient, Ecology, 97, 754–764, 2016.
Soliveres, S., van der Plas, F., Manning, P., Prati, D., Gossner, M. M., Renner, S. C., Alt, F., Arndt, H., Baumgartner, V., Binkenstein, J., Birkhofer, K., Blaser, S., Blüthgen, N., Boch, S., Böhm, S., Börschig, C., Buscot, F., Diekötter, T., Heinze, J., Hölzel, N., Jung, K., Klaus, V. H., Kleinebecker, T., Klemmer, S., Kraus, J., Lange, M., Morris, E. K., Müller, J., Oelmann, Y., Overmann, J., Pašalić, E., Rillig, M. C., Schaefer, H. M., Schloter, M., Schmitt, B., Schöning, I., Schrumpf, M., Sikorski, J., Socher, S. A., Solly, E. F., Sonneman, I., Sorkau, E., Steckel, J., Steffan-Dewenter, I., Stempfhuber, B., Tschapka, M., Tprke, M., Venter, P. C., Weier, C. N., Weisser, W. W., Werner, M., Westphal, C., Wilcke, W., Wolters, V., Wubet, T., Wurst, S., Fischer, M., and Allan, E.: Biodiversity at multiple trophic levels is needed for ecosystem multifunctionality, Nature, 536, 456–459, 2016.
Thebault, A., Mariotte, P., Lortie, C. J., and MacDougall, A. S.: Land management trumps the effects of climate change and elevated CO2 on grassland functioning, J. Ecol., 102, 896–904, 2014.
Theodorou, P., Baltz, L. M., Paxton, R. J., and Soro, A.: Urbanization is associated with shifts in bumblebee body size,with cascading effects on pollination, Evol. Appl., 14, 53–68, 2021.
Tilman, D., Fargione, J., Wolff, B., D'Antonio, C., Dobson, A., Howarth, R., Schindler, D., Schlesinger, W. H., Simberloff, D., and Swackhamer, D.: Forecasting agriculturally driven global environmental change, Science, 292, 281–284, 2001.
Vandewalle, M., De Bello, F., Berg, M. P., Bolger, T., Doledec, S., Dubs, F., Feld, C. K., Harrington, R., Harrison, P. A., Lavorel, S., da Silva, P. M., Moretti, M., Niemelä, J., Santos, P., Sattler, T., Sousa, J. P., Sykes, M. T., Vanbergen, A. J., and Woodcock, B. A.: Functional traits as indicators of biodiversity response to land use changes across ecosystems and organisms, Biodiv. Conserv., 19, 2921–2947, 2010.
Violle, C., Enquist, B. J., McGill, B. J., Jiang, L., Albert, C. H., Hulshof, C., Jung, V., and Messier, J.: The return of the variance: intraspecific variability in community ecology, Trends Ecol. Evol., 27, 244–252, 2012.
Wehner, K., Schuster, R., Simons, N. K., Norton, R. A., Blüthgen, N., and Heethoff, M.: How land-use intensity affects sexual and parthenogenetic oribatid mites in temperate forests and grasslands in Germany, Exp. Appl. Acarol., 83, 343–373, https://doi.org/10.1007/s10493-020-00586-z, 2021a.
Wehner, K., Renker, C., Simons, N. K., Weisser, W. W., and Blüthgen, N.: Trait filtering and narrow environmental niche predict land-use responses and vulnerability of land snail assemblages, BMC Evol. Biol., 21, 1–23, https://doi.org/10.1186/s12862-020-01741-1, 2021b.
Weiner, C. N., Werner, M., Linsenmair, K. E., and Blüthgen, N.: Land-use impacts on plant-pollinator networks: interaction strength and specialization predict pollinator declines, Ecology, 95, 466–474, 2014.
Wong, M. K. L. and Carmona, C. P.: Including intraspecific trait variability to avoid distortion of functional diversity and ecological inference: Lessons from natural assemblages, Methods Ecol. Evol., 12, 946–957, 2020.
Zhang, G., Han, X., and Elser, J. J.: Rapid top–down regulation of plant C: N: P stoichiometry by grasshoppers in an Inner Mongolia grassland ecosystem, Oecologia, 166, 253–264, 2011.